The work of the Quadrennial Technology Review 2015 is provided below, including links to the full report and to each of the individual chapters, links to the 51 technology assessments, and links to the 14 sets of Supplemental Information. Finally, a link to background information developed and released during the development of the QTR is provided at the bottom.
Technology Assessments
Chapter 3
[PDF] 3A Cyber and Physical Security
[PDF] 3B Designs, Architectures, and Concepts
[PDF] 3C Electric Energy Storage
[PDF] 3D Flexible and Distributed Energy Resources
[PDF] 3E Measurements, Communications, and Control
[PDF] 3F Transmission and Distribution Components
Chapter 4
[PDF] 4A Advanced Plant Technologies
[PDF] 4B Biopower
[PDF] 4C Carbon Dioxide Capture and Storage Value Added Options
[PDF] 4D Carbon Dioxide Capture for Natural Gas and Industrial Applications
[PDF] 4E Carbon Dioxide Capture Technologies
[PDF] 4F Carbon Dioxide Storage Technologies
[PDF] 4G Crosscutting Technologies in Carbon Dioxide Capture and Storage
[PDF] 4H Fast-spectrum Reactors
[PDF] 4I Geothermal Power
[PDF] 4J High Temperature Reactors
[PDF] 4K Hybrid Nuclear-Renewable Energy Systems
[PDF] 4L Hydropower
[PDF] 4M Light Water Reactors
[PDF] 4N Marine and Hydrokinetic Power
[PDF] 4O Nuclear Fuel Cycles
[PDF] 4P Solar Power
[PDF] 4Q Stationary Fuel Cells
[PDF] 4R Supercritical Carbon Dioxide Brayton Cycle
[PDF] 4S Wind Power
Chapter 6
[PDF] 6A Additive Manufacturing
[PDF] 6B Advanced Materials Manufacturing
[PDF] 6C Advanced Sensors, Controls, Platforms, and Modeling for Manufacturing
[PDF] 6D Combined Heat and Power Systems
[PDF] 6E Composite Materials
[PDF] 6F Critical Materials
[PDF] 6G Direct Thermal Energy Conversion Materials, Devices, and Systems
[PDF] 6H Materials for Harsh Service Conditions
[PDF] 6I Process Heating
[PDF] 6J Process Intensification
[PDF] 6K Roll-to-Roll Processing
[PDF] 6L Sustainable Manufacturing - Flow of Materials through Industry
[PDF] 6M Waste Heat Recovery Systems
[PDF] 6N Wide Bandgap Semiconductors for Power Electronics
Chapter 7
[PDF] 7A Bioenergy Conversion
[PDF] 7B Biomass Feedstocks and Logistics
[PDF] 7C Gas Hydrates Research and Development
[PDF] 7D Hydrogen Production and Delivery
[PDF] 7E Natural Gas Delivery Infrastructure
[PDF] 7F Offshore Safety and Spill Prevention
[PDF] 7G Unconventional Oil and Gas
Chapter 8
[PDF] 8A Connected and Automated Vehicles
[PDF] 8B Fuel Cell Electric Vehicles
[PDF] 8C Internal Combustion Engines
[PDF] 8D Lightweight Automotive Materials
[PDF] 8E Plug-in Electric Vehicles
Supplemental Information
Chapter 1
[PDF] Additional Information on Energy Challenges
[PDF] Agency Information
[PDF] DOE Energy Technology Roadmaps & Basic Research Needs
[PDF] Representative DOE Applied Energy Program Workshops
Chapter 5
[PDF] Building Technologies Office Potential Energy Savings Analysis
[PDF] Building Energy Technology Roadmaps
Chapter 6
[PDF] Competitiveness Case Studies
[PDF] Public-Private Consortia and Technology Transition Case Studies
Chapter 7
[PDF] Oil and Gas Technologies
[PDF] Subsurface Science, Technology, and Engineering
Chapter 9
[PDF] A Comparison of Research Center Funding Modalities
[PDF] High-Performance Computing Capabilities and Allocations
[PDF] User Facility Statistics
[PDF] Examples and Case Studies
More Information
General
Background Information on the Quadrennial Technology Review 2015
Quadrennial Technology Review 2011
2015 Quadrennial Technology Review
The 2015 Quadrennial Technology Review (QTR) examines the status of the science and technology that are the foundation of our energy system, together with the research, development, demonstration, and deployment (RDD&D) opportunities to advance them. I...
July 21, 2015Executive Summary
Introduction
The United States is in the midst of an energy revolution. Over the last decade, the United States has slashed net petroleum imports, dramatically increased shale gas production, scaled up wind and solar power, and cut the growth in electricity consumption to nearly zero through widespread efficiency measures. Emerging advanced energy technologies provide a rich set of options to address our energy challenges, but their large-scale deployment requires continued improvements in cost and performance. Technology is helping to drive this revolution, enabled by years to decades of research and development (R&D) that underpin these advances in the energy system.
The energy revolution underway creates additional opportunities for technologies and systems with superior performance and reduced costs. The convergence of many energy sectors—such as the electric grid, electricity production, buildings, manufacturing, fuels, and transportation—into systems linked through information and communications technologies (ICT), advanced modeling and simulation, and controls, has the potential to revolutionize energy services throughout the economy. These advances can enable the United States to address pressing national energy challenges—security, economic vitality, and climate change.
The 2015 Quadrennial Technology Review (QTR) examines the status of the science and technology that are the foundation of our energy system, together with the research, development, demonstration, and deployment (RDD&D) opportunities to advance them. It focuses primarily on technologies with commercialization potential in the midterm and beyond. It frames various trade-offs that all energy technologies must balance across such dimensions as cost, security and reliability of supply, diversity, environmental impacts, land use, and materials use. Additionally, it provides data and analysis on RDD&D pathways to assist decision makers as they set priorities, within budget constraints, to develop more secure, affordable, and sustainable energy services. Policies and regulations are examined separately by the Quadrennial Energy Review (QER).
National Energy System Strategic Objectives
Three enduring strategic objectives are foundational to our nation’s energy system: energy security, economic competitiveness, and environmental responsibility.
Secure and resilient: There are four interrelated dimensions to energy security: physical, cyber, supply, and conflict-related. Physical security risks are related to damage to energy supply, storage, and delivery infrastructures, such as the electric grid, pipeline networks, and rail and marine systems. Cybersecurity vulnerabilities are related to the compromise of ICT-based controls that operate and coordinate energy supply, delivery, and end-use systems. Supply security risks are related to price shocks and international supply disruptions of energy commodities, critical materials, and/or equipment. Conflict–related security risks are associated with unrest in foreign countries linked to, or impacting, energy. Climate change increases physical security risks with sea level rise and intensification of extreme weather.
Economically competitive: Energy underpins every facet of the nation’s economy and modern way of life. Low energy costs are beneficial to consumers and therefore the broader economy. Decades of research have gone into reducing the capital, operating, and fuel costs of conventional and advanced energy technologies. The benefits of this research are evident in the recent price declines of natural gas, domestic oil, wind turbines, photovoltaics, and efficient lighting. Progress in a broader array of advanced technologies could increase the diversity and stability of energy supplies, and spark competition to drive further price declines.
Environmentally responsible: Development of a clean energy system will rely on increasingly advanced technologies to minimize its environmental footprint. Over the last several decades, the United States has made significant progress in reducing pollution—atmospheric, water, land—from energy-related activities. For example, energy-related atmospheric emissions of conventional pollutants such as particulates, sulfur, and nitrogen compounds have been reduced through improved combustion strategies and “end-of-pipe”—e.g., scrubbers, catalytic converters—emissions controls. Additional emissions reductions have been achieved by improving efficiency and transitioning to cleaner fuels and low-carbon resources. These successes demonstrate what can be accomplished with RDD&D and policy. Advanced technologies can have a significant impact on the next generation of challenges, especially deep reductions in greenhouse gas (GHG) emissions to moderate the otherwise increasing damage from climate change and ocean acidification. The United States can demonstrate the viability of sustainable energy systems to the global community and provide leadership in creating vibrant economies, enhancing human progress, and assuring a sustainable biosphere.
Developing energy systems that balance trade-offs to simultaneously advance toward these objectives requires RDD&D across a diversified portfolio of technologies. It also requires understanding the multiple dimensions of each of these objectives.
Energy System Perspective
To help identify where RDD&D can have the greatest impact, it is first necessary to understand how energy is used in the United States. A complex and vast array of systems and associated technologies extract energy resources; convert them into usable forms of energy; and deliver them to end users to provide desired services such as manufactured goods, thermal comfort, lighting, and mobility. The current state of energy supplies and end uses is described in Chapter 1.
Increasing the interconnectedness and resulting interdependency among energy sectors creates both opportunities and challenges that should be approached from an energy system perspective. Strategies for advancing technology across the entire energy system, in contrast to individual energy technologies, are necessarily broad.
First, certain technologies affect the energy system by impacting more than one energy sector. Application of an energy system view of technology can help to identify the crosscutting impacts of technologies developed for a particular application as applied to other sectors, as well as the elements of the value chain that must be in place for success. Realizing the full benefit of developing these crosscutting technologies requires the involvement of stakeholders from across the energy economy.
Second, the systems perspective can illuminate opportunities to improve performance and/or mitigate risk through sector integration. Success requires advancing the operation, planning, modeling, and simulation of technical systems integrated across sectors.
Finally, application of an energy system view can be used to develop solutions to complex energy challenges. New paradigms based on the science of large and complex systems can help enable the prediction and control of emergent properties and behaviors, including disruptions that arise from sector and technical system interconnectedness. The focus of Chapter 2 is Energy Sectors and Systems.
Overarching Themes, Energy Sectors, and Crosscutting RDD&D Opportunities
By studying the whole energy system and the interdependency of the energy sectors, four overarching themes, six sets of core RDD&D opportunities (organized by energy sector), and twelve crosscutting technology areas are identified and presented.
Overarching Themes
Four overarching themes emerged from the QTR and associated technology assessments: 1) the convergence of energy systems across sectors; 2) diversification within energy supplies and services; 3) confluence of R&D, computational tools, and analysis of complex systems; and 4) energy efficiency.
Convergence: Virtually all sectors of the energy system are becoming increasingly interdependent. Further, the power, grid, buildings, manufacturing, fuels, and transportation sectors of the energy system are necessarily coupled to water systems, material flows, waste products, and energy financial markets. Properly tuned and integrated energy sectors and technology systems have the potential to improve their overall operations, increase their efficiency, and enable fundamentally new concepts in the structure of the energy economy.
Diversification: Most energy sectors in the United States are experiencing a trend toward increased diversification. For example, electricity, hydrogen, natural gas, and biofuels are entering the transportation sector, while the power sector is shifting to greater use of natural gas and renewables. This diversification creates challenges for energy infrastructures, but it can also provide flexibility.
Confluence: The confluence of advances in computing power and software, theory, modeling, synthesis and characterization is rapidly ushering in a new era of “systems by design” for materials, chemicals, and biological science. This transformation from observation to control and design of complex systems has the potential to accelerate development of these systems with desired properties. This set of concepts—generalized to new classes of sensors, big data management, and computational modeling—is applicable across the spectrum of complex systems topics encountered in the energy system.
Efficiency everywhere: Achieving greater efficiency is a proven means to help achieve national energy security, cost, and environmental goals. As raw energy resources are transformed into services and products, losses compound along the energy value chain. Efficiency improvements in any step along the value chain can materially impact costs, consumption, and emissions. RDD&D opportunities to advance cost-effective efficiency technologies permeate all of the energy sectors and systems.
Energy Sectors
The QTR describes the national energy system as comprising six individual sectors: 1) the electric grid, 2) electricity production (power), 3) buildings (residential and commercial), 4) manufacturing (the majority of the larger industrial sector), 5) fuels (with an emphasis on fuels for transportation), and 6) transportation. Each of these sectors comprises numerous technical systems, sub-systems, and component technologies. The QTR dedicates a chapter to each of these six sectors, exploring its related technologies, challenges, and RDD&D opportunities.
Figure ES.1 Sankey Diagram of the U.S. Energy System Depicting Major Areas of Coverage by the Technical QTR Chapters 3–8
Electrical grid sector: The U.S. electric power sector is the centerpiece of the nation’s energy economy. However, the design and operation of today’s grid is being challenged to meet the evolving security, cost, and environmental needs of a low-carbon, digital economy. Shifts are occurring on the supply side (e.g., increased adoption of renewable resources) and demand side (e.g., growing use of demand side management). Accompanying these changes is the growing adoption of digital communications and control systems (i.e., smart grid technologies) to improve performance and engage consumers. Additionally, grid operation is moving from controlling systems with a handful of control points at central stations to ones with potentially millions of highly interactive distributed control points. In short, the power grid is confronted with new requirements as it attempts to perform in ways for which it was not designed. Meanwhile, the nation’s reliance on a dependable, efficient, and resilient power grid is rising. The focus of Chapter 3 is Enabling Modernization of the Electric Power System.
Electricity production sector: The current portfolio of electricity production includes a combination of reliable but aging base-load generation, evolving renewable resources, new natural gas plants, and new and pending nuclear and clean coal facilities. As the industry evolves to meet growing electrification and GHG reduction goals, challenges arise in optimizing the system, minimizing risks, and maintaining reasonable cost. Future developments will likely include a mix of three broad categories: 1) fossil-based generation with carbon capture and storage (CCS), 2) nuclear energy, and 3) renewables, such as solar and wind. Technologies that enable higher efficiencies and effective pollution control are an essential complement to this evolving generation mix. Similarly, crosscutting concepts—such as supercritical carbon dioxide Brayton cycles—could, if broadly applied, impact efficiency, emissions, and water consumption across fossil, nuclear, geothermal, and solar thermal plants. While supporting aggressive emission reductions, the traditional market drivers such as reliability, safety, and low cost must be maintained and enhanced. The focus of Chapter 4 is Advancing Clean Electric Power Technologies.
Buildings sector: Considerable potential exists to reduce building energy use. The residential and commercial buildings sector accounts for about 74% of electricity use and 40% of all U.S. primary energy use. Many building technologies are available today that would significantly reduce energy use relative to the existing building stock. Yet, the best available and most cost effective ones are only beginning to be widely adopted in the marketplace. It has become increasingly apparent that technology developments in the buildings sector have the potential to simultaneously accelerate cost reductions, service improvements, and efficiency gains. Advanced heating/cooling and lighting are current R&D priorities, as they represent the greatest end-use energy-saving opportunities. Much progress is being made in areas such as light-emitting diode (LED) lighting, appliances, and non-vapor compression heating, ventilation, and air conditioning (HVAC). Miscellaneous electric loads and an eclectic mix of technologies (e.g., grills, spa and pool pumps, laundry, and elevators) are expected to be an increasing share of the remaining load as other end uses become significantly more efficient. The focus of Chapter 5 is Increasing Efficiency of Building Systems and Technologies.
Manufacturing sector: Manufacturing consumes twenty-four quads of primary energy annually in the United States—about 79% of total industrial energy use. However, this sector’s energy impacts are much broader, as manufactured goods affect the production, delivery, and use of energy across the economy. Improved manufacturing technologies can drive economy-wide energy impacts, including energy efficiency in the manufacturing sector; new types of manufactured products; and sustainability of U.S. industry supply chains and their life-cycle impacts. The focus of Chapter 6 is Innovating Clean Energy Technologies in Advanced Manufacturing.
Fuels Sector: Fuels supply 99.8% of the energy currently used in the transportation sector and 70% of the energy used to generate electricity in the United States. The economy will need to balance the various strengths and shortcomings of a broad mix of fuels during the transition from a high-carbon to a low-carbon economy. This fuel mix includes the following:
- Fossil fuels: Chemical fuels, primarily derived from fossil energy resources, supply about 83% of total U.S. primary energy use.
- Bioenergy for fuels: With technology improvement and a mature market, available bioenergy could provide more than fifty billion gallons of fuels per year, equivalent to about 25% of current transportation fuel demand.
- Hydrogen production and distribution: Technologies for producing hydrogen from large natural gas reforming plants are mature, but the costs of converting the end-to-end fuels infrastructure, including delivery, to accommodate hydrogen are high. While the near-term deployment challenge is to reduce the cost of infrastructure for fueling vehicles, in the longer term the major challenge is to reduce the cost of hydrogen production from regionally optimized renewable and low-carbon resources.
With recent growth in domestic shale gas and tight oil production, near-term concerns over fuel supply and energy security are easing. However, the economic and environmental impacts of heavy reliance on fossil fuels make their further cleanup or transition to clean alternatives imperative. The trade-offs between conventional (oil and gas) and alternative fuels (primarily biofuels and hydrogen) or substitution with electricity—i.e., cost, performance, infrastructure, security, and environmental impacts—are complex. Optimizing the benefit of fuel diversification is challenged by the varying time frames for development and deployment of fuels, production and distribution infrastructures, and end-use devices such as vehicles. The focus of Chapter 7 is Advancing Systems and Technologies to Produce Cleaner Fuels.
Transportation Sector: Transportation provides essential passenger, freight, and other mobility services to individuals and the economy. It is the primary user of petroleum in the United States and a major emitter of air pollutants and GHGs. Currently, light- and heavy-duty vehicles account for approximately three-quarters of transportation energy use and emissions. Other modes in the transportation system include rail, marine, aircraft, and pipelines, the proportional emissions from which are likely to grow in importance as the efficiency of on-road transportation technologies improves. To greatly reduce GHG emissions, a larger share of vehicles must efficiently use fuels or power with drastically reduced life-cycle carbon emissions. The technology portfolio benefits from a set of complementary RDD&D pathways, including advanced combustion, light-weighting, battery storage, electric drivetrain, fuel cell systems, and recharging and refueling infrastructure. Addressing the transportation sector as a holistic system that encompasses more than just vehicle technologies is another important emerging research opportunity. The focus of Chapter 8 is Advancing Clean Transportation and Vehicle Systems and Technologies.
Cross-Cutting RDD&D Opportunities
Inevitably, many technology themes were identified that cut across the six sectors. As a result, they should be integrated in ways that bridge strict sectoral boundaries. In a simplified view, the crosscutting topics can be grouped into two major categories: “technical topics” and “enabling tools.”
The “technical topics” include the following:
- Grid modernization: Advanced grid technologies are needed to improve the agility and flexibility of the system to better integrate the changing characteristics of devices and technology systems on both the supply and demand sides.
- Systems integration: Appropriate application of systems integration requires understanding, control, and optimization across multiple sectors, time frames, and spatial scales. An integrated systems approach can address complexity and enable more efficient deployment of advanced energy technologies.
- Cybersecurity:Opportunities to improve cybersecurity are being actively pursued for the energy sector (i.e., electric, oil, and gas), and also exist in industrial automation systems and information technologyenabled innovations across the fuels, power generation, buildings, manufacturing, and intelligent vehicle spaces.
- Energy-water:Science and technology advancements at the intersection of energy and water can reduce energy use and increase water availability for human consumption, other non-energy uses, and natural systems.
- Subsurface: Understanding and controlling fractures, fluid flow, and complex chemistry in subsurface rock formations on timescales of microseconds to millennia are important for oil and gas production, geothermal energy, CCS, and nuclear waste disposal.
- Materials: Across all energy sectors, advancements in materials could dramatically accelerate and reduce the cost of developing new energy technologies. Examples include development of materials for extreme working conditions, advanced processing of them, and their rapid qualification.
- Fuel-Engine co-optimization: With bio-derived and/or other synthetic fuels there is an opportunity to optimize the end-to-end fuel-vehicle system for improved efficiency and reduced environmental impacts.
- Energy storage: Fundamental research on efficient, durable storage could enable transformational change across multiple sectors, including transportation, and the electricity system.
The “enabling tools” include the following:
- Computational modeling and simulation: Advances in high performance computation have enabled simulation of increasingly complex physical phenomena. High-fidelity simulations, in turn, inform models that improve and accelerate the RDD&D phases of the energy innovation cycle.
- Data and analysis:Opportunities to apply advanced analytics transect the entire clean energy economy. The emerging science of extracting actionable information from large data sets is both an opportunity to accelerate RDD&D and a research need.
- Analysis of complex systems: Increasing complexity resulting from the convergence of the sectors of the energy system introduces a need for foundational, conceptual research on integrated, networked, and complex systems.
- Characterization and control of matter at multiscales: Extraordinary advances in characterization and modeling of materials and chemistry have paved the way for manipulating and synthesizing materials at the atomic-, nano-, and mesoscale to create new tailored functionalities. The research spans a range of dimensions from the atom, to biological cells, to macroscopic structures, with applications across many scientific and engineering disciplines.
The crosscutting RDD&D opportunities listed here offer the potential to dramatically improve the performance and posture of all energy resources and end uses. They represent a condensed set of concepts—linked by an overarching goal to understand, predict, and control complex energy systems—that appear in more than one of the technology areas of this report (see Table 2.1).
Enabling Capabilities for Science and Energy
Investment in basic science research is expanding our understanding of how structure leads to function—from the atomic- and nano-scale to the meso-scale and beyond—and is enabling a transformation from observation to control and design of new systems, with properties tailored to meet the requirements of the next generation of energy technologies. The challenges in energy science and technology development increasingly necessitate interdisciplinary collaboration. The multidisciplinary and multi-institutional research centers supported by DOE and others have the potential to accelerate development of new and transformative energy technologies by more effectively integrating basic science and applied research. At the core of this new paradigm is a diverse suite of complex experimental and computational tools that enable researchers to probe and manipulate matter at unprecedented resolution. The planning for and development of these tools are rooted in basic science, but they are critically important for technology development, enabling discoveries that can lead to broad implementation. These tools are available through a user facility access model that provides open access, regardless of institutional affiliation, for nonproprietary research based on merit review of submitted proposals. This is a synergistic model: thousands of scientists and engineers leverage the capabilities and staff expertise for their research, while the facilities leverage user expertise toward maintenance, development, and application of the tools in support of the broader community of users. The focus of Chapter 9 is Enabling Capabilities for Science and Energy.
Concepts in Integrated Analysis
A goal of technology development programs, whether in the private sector or in government institutions, is to maximize the positive impact of RDD&D portfolio investments in energy technologies. The many technologies described in this QTR illustrate the potential impacts that further RDD&D could have to create a secure, competitive, and clean energy system. Weighting of these impacts, as well as the metrics from which they are built (e.g., cost, performance, land use, water quality, GHG emissions, etc.), will necessarily vary with the perspective of the observer. Research institutions must consider multiple impact metrics that address their overarching goals from a business or public perspective. To this end, portfolio analysis is widely employed, but at varying levels of thoroughness, analytic rigor, and transparency. Many tools for technology planning and projection, analysis, metrics calculation, and impact evaluation exist already, but are not necessarily fully developed or packaged in a way that can be readily used for evaluating energy portfolios. This QTR explores processes to shape an energy portfolio and estimate the potential impacts, articulates the current state of integrated technology assessment, gives examples of sector-specific applications of metrics and tools for technology analysis in use in various organizational contexts (i.e., corporate, nonprofit, academic, and government), and identifies gaps that require further development of technical assessment capabilities. The focus of Chapter 10 is Concepts in Integrated Analysis.
Conclusion
The world of energy-related research is rich with opportunities to help create a secure, resilient, economically efficient, and environmentally responsible set of energy systems. Those systems will rely on more efficient energy conversion technologies and will benefit from improved understanding of complex, interdependent systems that provide electricity, transportation, water, and materials for manufacturing. Underlying the advances in those areas will be the many technologies and capabilities described in this report, as well as fundamental scientific research and advanced scientific computing for complex systems. The technology development community is beginning to take advantage of the rapidly emerging set of tools for creating new generations of materials, devices, and systems for energy applications; however, much more can be done. A goal is to put these new tools in their hands to drive a well-diversified portfolio of energy research that will enable leadership by the United States to provide the energy services essential to modern societies.
The introductory chapter of the 2015 Quadrennial Technology Review frames the R&D opportunities, explored in the remainder of the report, in context. It summarizes the state of the system, recent changes, strategic objectives and fundamental challenges.
July 21, 2015Chapter 1 — Energy Challenges
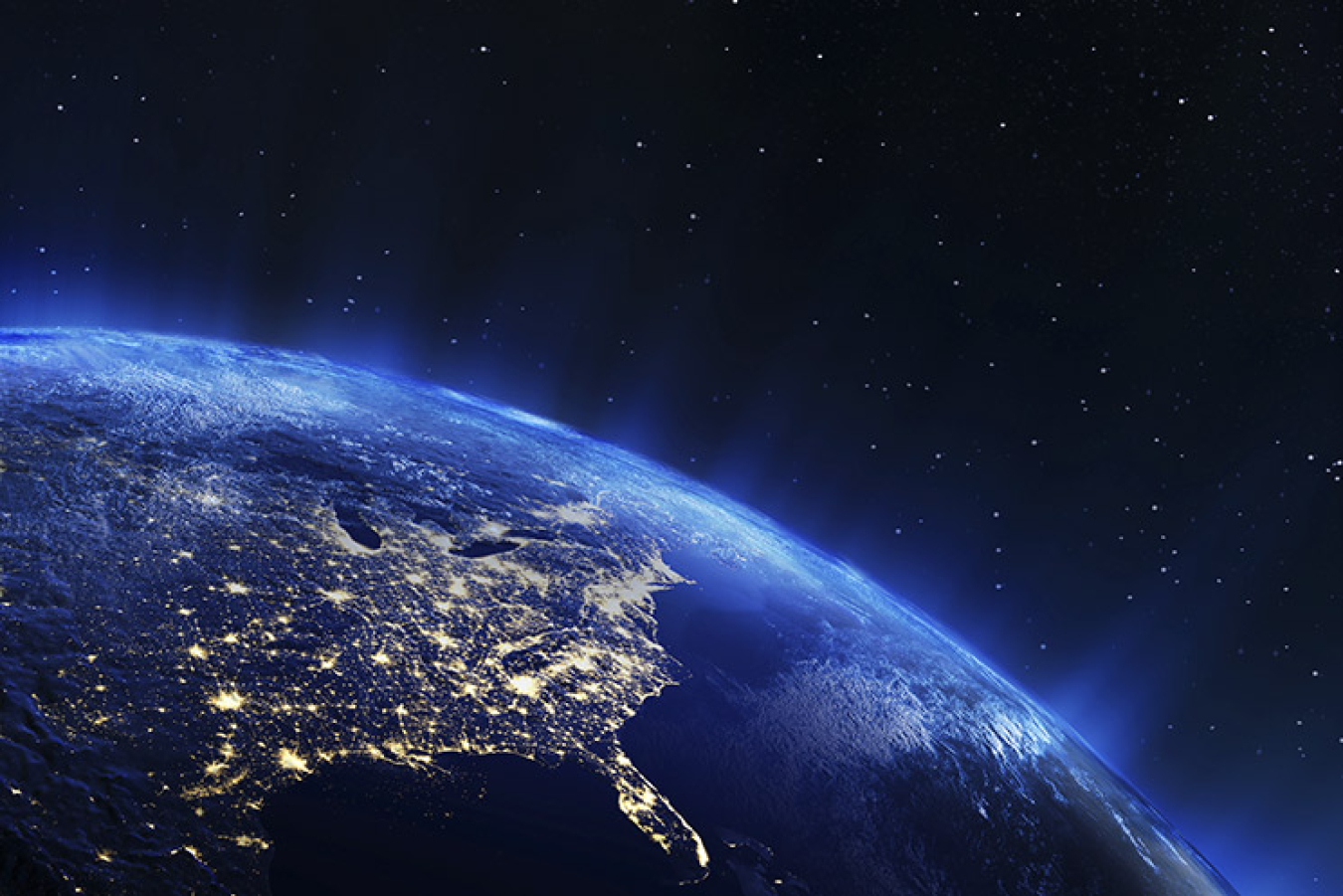
The United States’ energy system, vast in size and increasingly complex, is the engine of the economy. The national energy enterprise has served us well, driving unprecedented economic growth and prosperity and supporting our national security. The U.S. energy system is entering a period of unprecedented change; new technologies, new requirements, and new vulnerabilities are transforming the system. The challenge is to transition to energy systems and technologies that simultaneously address the Nation’s most fundamental needs—energy security, economic competitiveness, and environmental responsibility—while providing better energy services.
The introductory chapter of the 2015 Quadrennial Technology Review frames these energy challenges and the R&D opportunities, explored in the remainder of the report. It summarizes the state of the system, recent changes, and strategic objectives.
This chapter presents a holistic view of the energy system and explores the opportunities in energy systems research. Systems approaches can help to identify critical technology needs and can also be used to develop solutions to complex energy challen...
July 21, 2015Chapter 2 — Energy Sectors and Systems
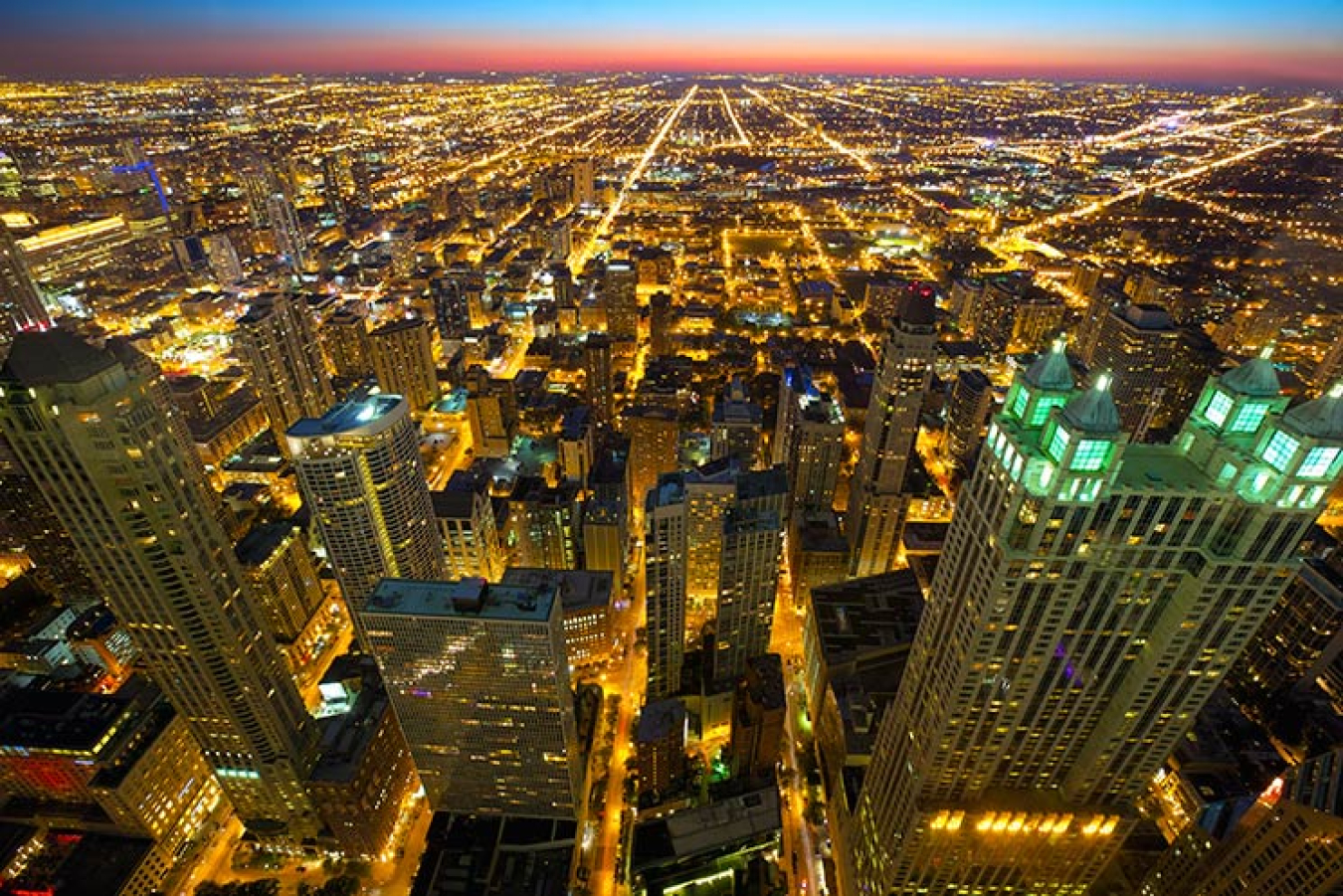
Within and between the electricity, fuels, transportation, buildings, and manufacturing sectors, increasing interconnectedness and complexity are creating opportunities and challenges that can be approached from a systems perspective. Some of the most transformational opportunities exist at the systems level. They are enabled by the ability to understand, predict, and control very large scale and interconnected complex systems. Systems solutions can be broadly impactful across multiple technologies and sectors.
This chapter presents a holistic view of the energy system and explores the opportunities in energy systems research. Systems approaches can help to identify critical technology needs and can also be used to develop solutions to complex energy challenges.
The electric power system is facing increasing stress due to fundamental changes in both supply and demand technologies. Advances in the power system are needed to address these issues and ensure system reliability. The research needs that can help rea...
July 21, 2015Chapter 3 — Enabling Modernization of the Electric Power System
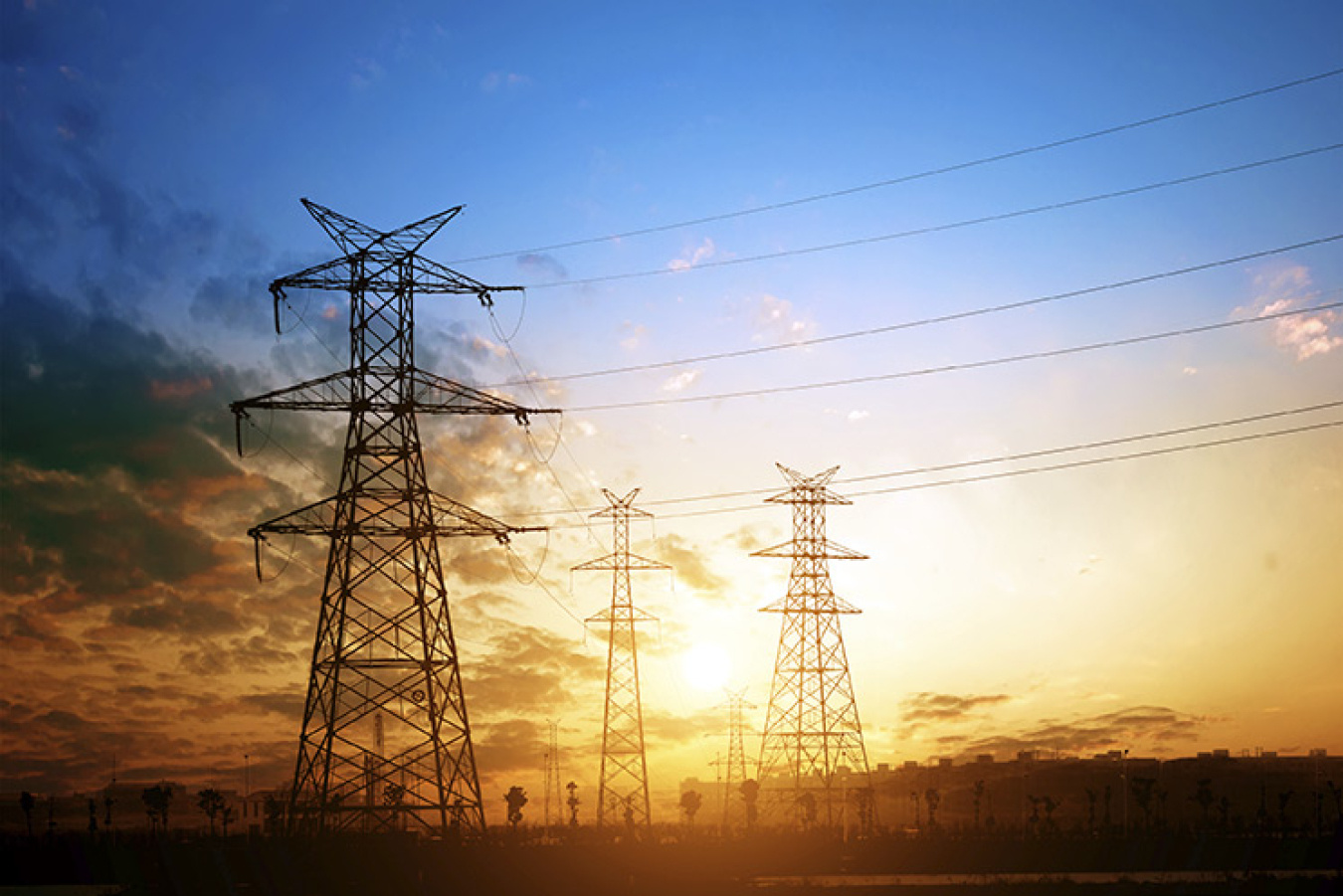
The electric power system is facing increasing stress due to fundamental changes in both supply and demand technologies. On the supply side, there is a shift from large synchronous generators to lighter-weight generators (e.g., gas-fired turbines) and variable resources (renewables). On the demand side, there is a growing number of distributed and variable generation resources as well as a shift from large induction motors to rapidly increasing use of electronic converters in buildings and, industrial equipment. The communications and control systems are also transitioning from analog systems to systems with increasing digital control and communications; from systems with a handful of control points at central stations to ones with potentially millions of control points.
With these and other changes, the system is being asked to perform in ways and in a context for which it was not designed. The result is a system that is under increasing stress from these and other factors and requires much greater flexibility, agility, and ability to dynamically optimize grid operations in time frames that are too fast for human operators. Fundamental advances in the power system are needed to address these changes and ensure system reliability. These changes, however, also open a set of opportunities that can be tapped to significantly improve performance, lower costs, and address our national energy challenges. The research needs that can help realize these opportunities are described in this chapter.
This chapter describes the current status and future outlook for power generation technologies, and identifies RDD&D directions that will contribute to a portfolio of technology options that can meet future regional demands. A combination of flexible ...
July 21, 2015Chapter 4 — Advancing Clean Electric Power Technologies
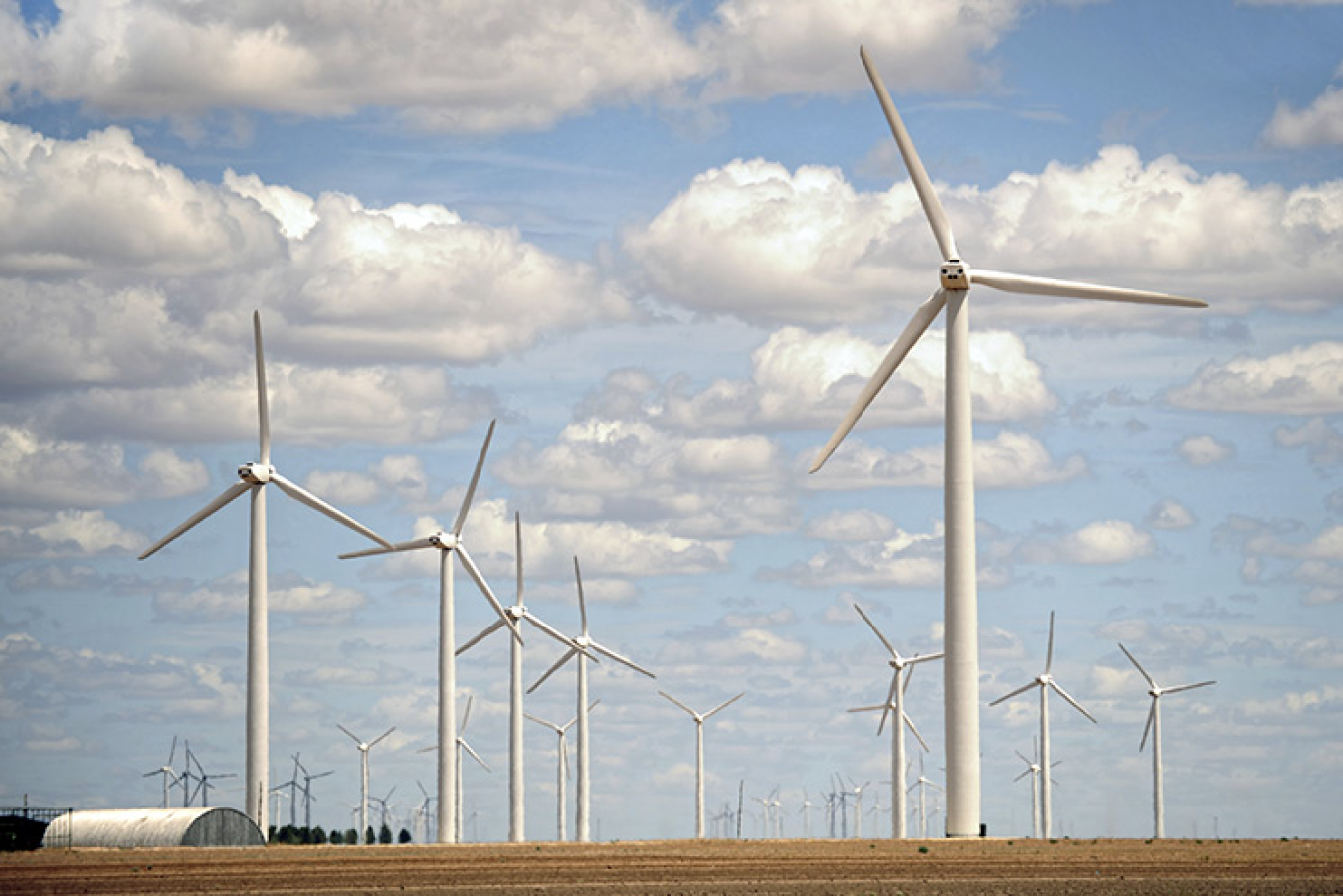
Clean electric power is paramount to today’s mission to meet our interdependent security, economic, and environmental goals. While supporting aggressive emission reductions, the traditional market drivers such as reliability, safety, and affordability must be maintained and enhanced. The current portfolio of electric production includes a combination of coal, nuclear (with five new reactors under construction), hydro, growing natural gas, and rapidly advancing renewable generation sources. Complementing this evolving generation mix, technologies to enable higher efficiencies, pollution control, and carbon capture and storage are essential aspects of the RDD&D portfolio.
This chapter describes the current status and future outlook for power generation technologies, and identifies RDD&D directions that will contribute to a portfolio of technology options that can meet future regional demands. A combination of flexible technology options will be required to meet increasing power needs in the U.S. and globally. The QTR focuses on technological advances to meet U.S. energy needs and challenges, recognizing that these also offer opportunities for cooperative research that will expedite the international deployment of these technologies.
This chapter describes the next generation of research opportunities in building energy technologies.
July 21, 2015Chapter 5 — Increasing Efficiency of Buildings Systems and Technologies
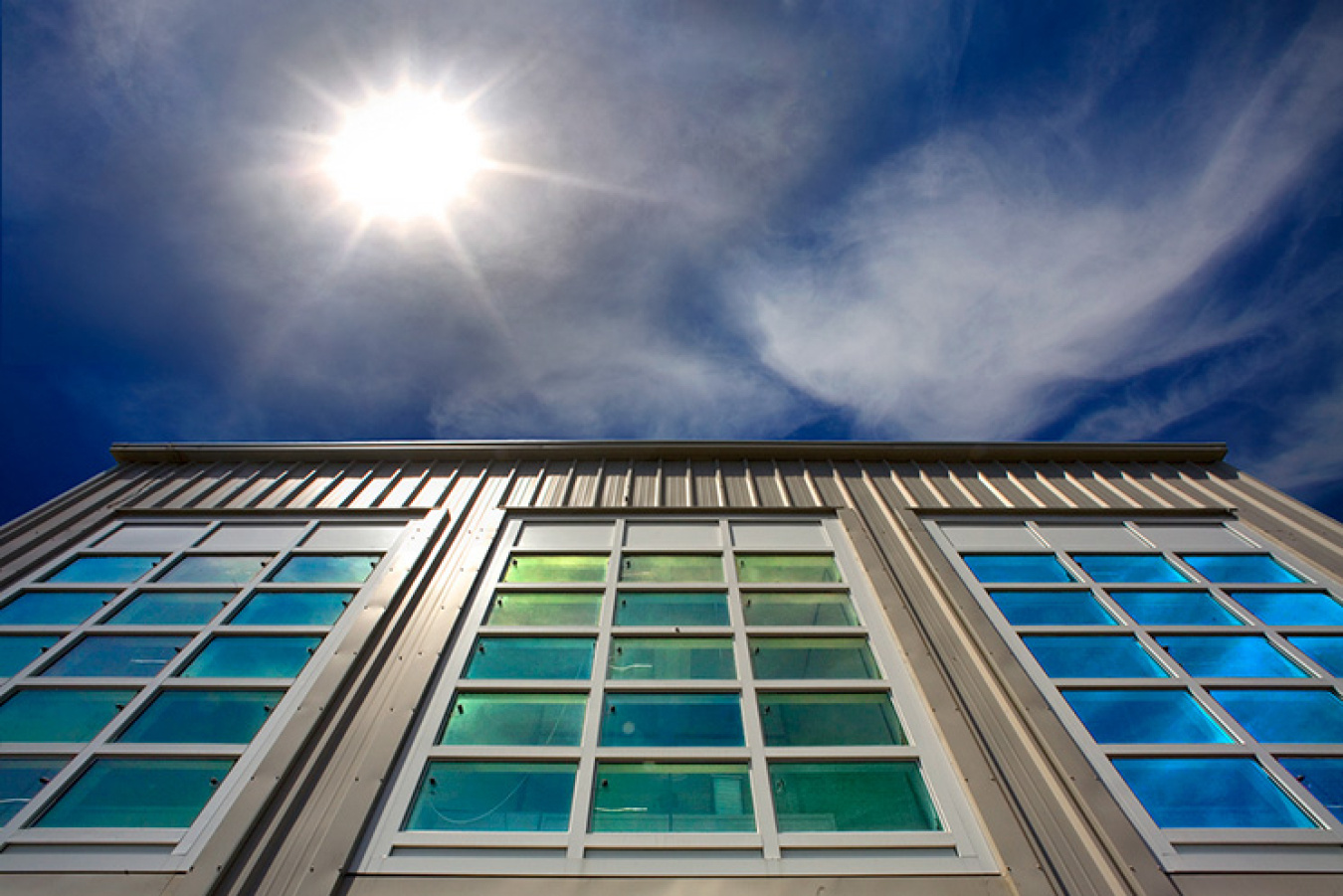
The buildings sector accounts for about 76%* of electricity use and 40% of all U.S. primary energy use and associated greenhouse gas (GHG) emissions, making it essential to reduce energy consumption in buildings in order to meet national energy and environmental challenges and to reduce costs to building owners and tenants. Opportunities for improved efficiency are enormous. By 2030 building energy use could be cut more than 20% using technologies known to be cost effective today and by more than 35% if research goals are met. Much higher savings are technically possible.
Building efficiency must be considered as improving the performance of a complex system designed to provide occupants with a comfortable, safe, and attractive living and working environment. This requires superior architecture and engineering designs, quality construction practices, and intelligent operation of the structures. Increasingly operations will include integration with sophisticated electric utility grids.
The major areas of energy consumption in buildings are heating, ventilation, and air conditioning; lighting, major appliances (water heating, refrigerators and freezers, dryers); and a significant fraction remaining in miscellaneous areas including electronics. In each case there are opportunities both for improving the performance of system components and improving the way they are controlled as a part of integrated building systems.
This chapter describes the next generation of research opportunities in building energy technologies.
*Including space heating and lighting industrial buildings
This chapter examines the opportunities for improvements in energy and materials utilization within three spaces: individual manufacturing processes and unit operations; goods-producing facilities, including manufacturing business processes; and manufa...
July 21, 2015Chapter 6 — Innovating Clean Energy Technologies in Advanced Manufacturing
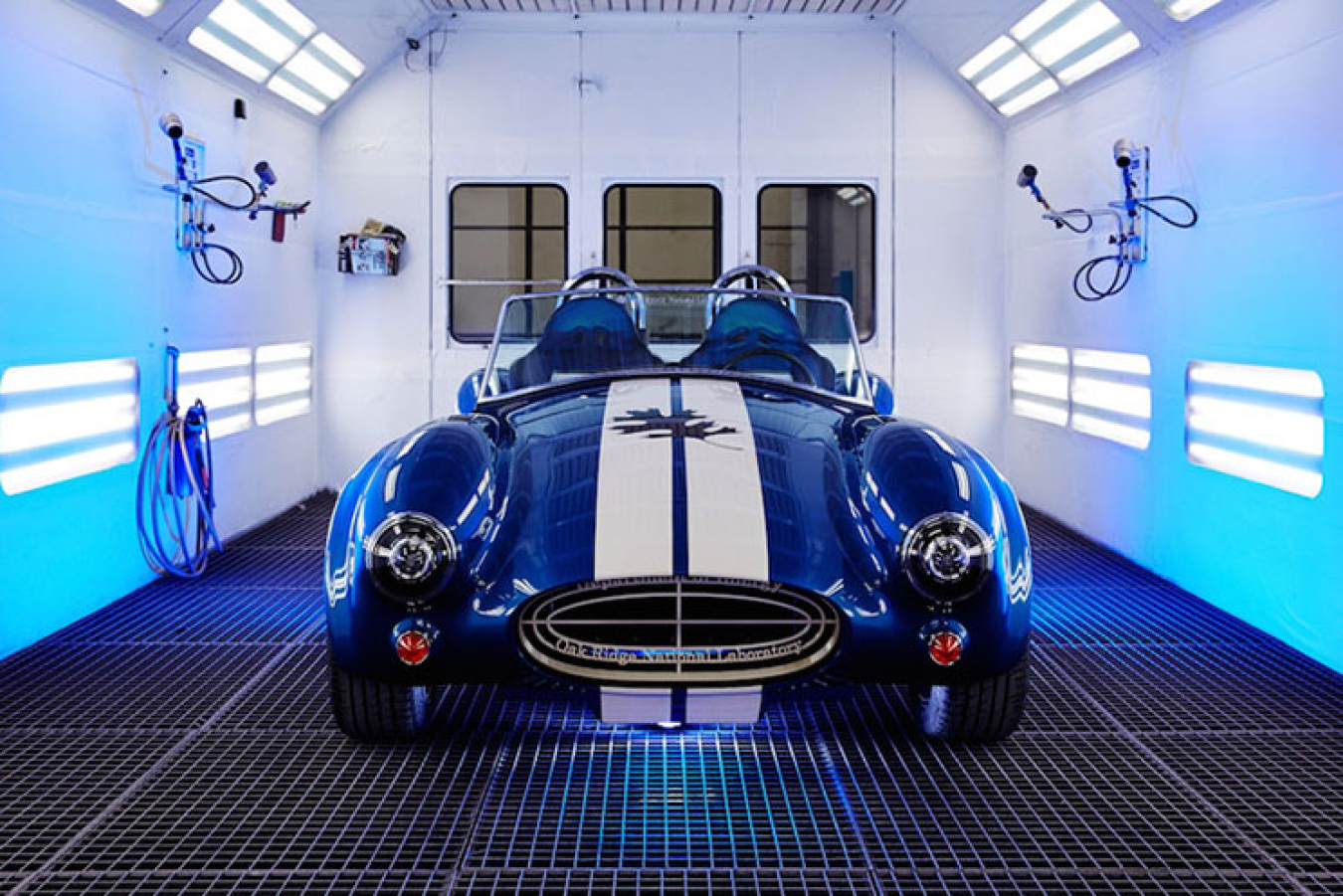
Clean energy manufacturing involves the minimization of the energy and environmental impacts of the production, use, and disposal of manufactured goods, which range from fundamental commodities such as metals and chemicals to sophisticated final-use products such as automobiles and wind turbine blades. The manufacturing sector, a subset of the industrial sector, consumes 24 quads of primary energy annually in the United States. Clean energy manufacturing will improve energy utilization and also yield economy-wide reductions in greenhouse gas (GHG) emissions through changes in energy use enabled by the development of new materials and process technologies.
This chapter examines the opportunities for improvements in energy and materials utilization from three perspectives: individual manufacturing processes and unit operations; goods-producing facilities; and manufacturing supply chains, including energy impacts from all phases of the product life cycle for manufactured goods.
This chapter examines the opportunities for improvements in energy and materials utilization within three spaces: individual manufacturing processes and unit operations; goods-producing facilities, including manufacturing business processes; and manufa...
July 21, 2015Chapter 7 — Advancing Systems and Technologies to Produce Cleaner Fuels
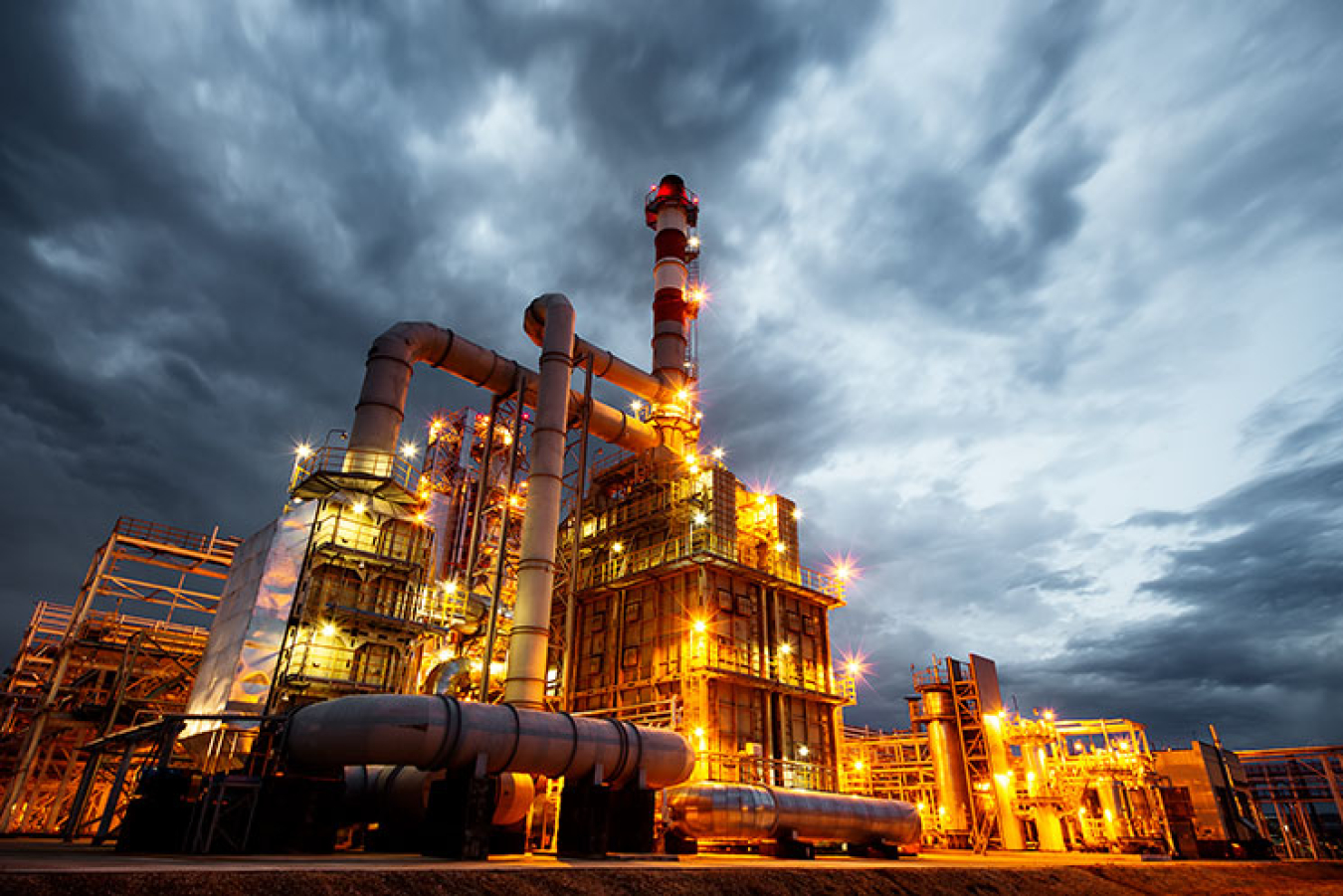
Fuels play a critical role throughout our economy. In 2013, fuels directly supplied about 99% of the energy needed by our national transportation system, 66% of that needed to generate our electricity, 68% of that needed by our industry, and 27% of that needed by our buildings. For the purposes of this Quadrennial Technology Review (QTR), a “fuel” is defined as a carrier of chemical energy that can be released via reaction to produce work, heat, or other energy services. Fuel resources include oil, coal, natural gas, and biomass. The source and mix of fuels used across the sectors of the energy system is changing, particularly the rapid increase in production from unconventional oil and gas resources.
This chapter considers three primary fuel pathways—oil and natural gas, biomass, and hydrogen, their associated technology and industrial ecosystems, and the economic, security, and environmental challenges they face. For each, current technology is reviewed and key RDD&D opportunities are identified that could help resolve their challenges. In the oil and gas sector, the primary Federal role in research is related to prudent development of these resources. Biofuels require RDD&D across the entire value chain, from resources through conversion to a variety of refined products. Hydrogen can be produced via a variety of industrially proven technologies from fossil sources such as natural gas, but further RDD&D is needed to reduce the cost of producing hydrogen from renewable, low-carbon resources. Hydrogen’s other challenges include on-board storage cost, lack of transmission and distribution infrastructure, and fuel cell cost and durability, as well as economic scale-up across the entire value chain. The chapter concludes with a brief survey of additional fuel pathways (coal and biomass to liquids, dimethyl ether, ammonia, etc.), each of which has intrinsic technological merit, but all of which also face challenges.
This chapter focuses mainly on technologies applicable to light and heavy duty road vehicles, and surveys other modes as well as systems-level technologies for the improvement of energy use across the transportation system.
July 21, 2015Chapter 8 — Advancing Clean Transportation and Vehicle Systems and Technologies
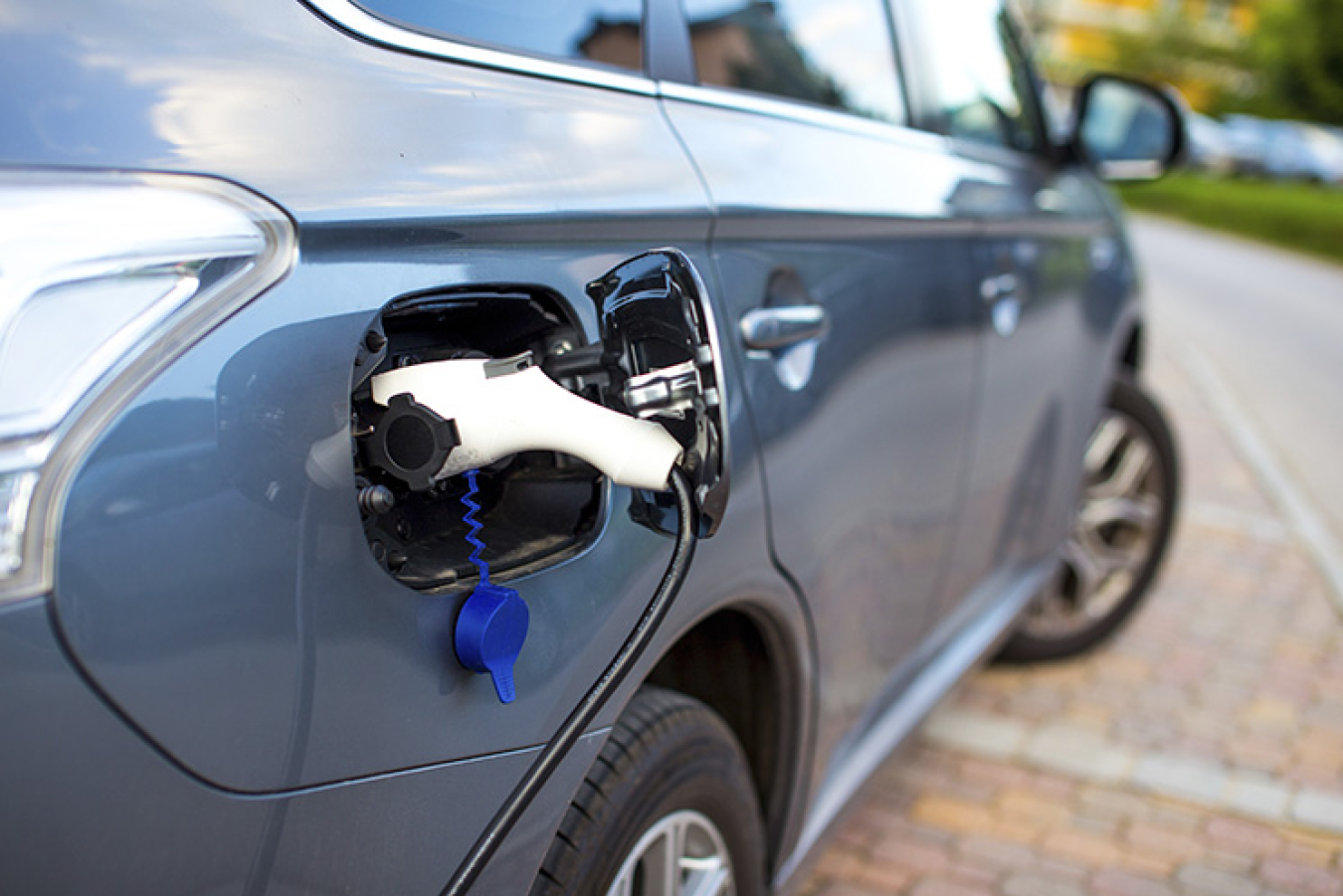
Transportation is a complex sector composed of light duty, medium duty, heavy duty, and non-highway vehicles; rail; aircraft; and ships used for personal transport, movement of goods, construction, agriculture, and mining as well as associated infrastructure. Transportation accounts for approximately 10% of gross domestic product (GDP) and depends on significant public sector investment for construction and maintenance of roads, traffic management, transit, airports, ports, and waterways. Transportation uses 27 quadrillion British thermal units (Btu) of petroleum annually, representing 70% of U.S. petroleum use, and 92% of energy for transportation is from petroleum, which means that any strategy to improve our economic and energy security by reducing our dependence on petroleum must include transportation.
The need for a safe, energy-efficient, operationally efficient, low emission, and flexible transportation system can be addressed by advancing technology throughout the transportation system. The need to address energy security concerns, oil import costs, and emissions has long been a national priority driving the Department of Energy’s (DOE) transportation technology research on efficient vehicle drivetrains, including efficient combustion vehicles, plug-in electric vehicles, and fuel cell vehicles. Future personal vehicle markets—in which these efficient vehicle technologies compete—may be transformed by information technologies as well as by social and demographic trends. Technologies, including plug-in electric vehicles and fuel cell vehicles, offer the potential for integration between other energy systems such as electricity and buildings, which could be pursued to improve economy-wide efficiency and reduce emissions. This chapter focuses mainly on technologies applicable to light and heavy duty road vehicles, and surveys other modes and systems-level technologies considerations that could improve energy use across the transportation system.
This chapter is a survey of how the Department of Energy (DOE) and the DOE-SC support energy technology through investment in basic science research and development of complex and unique experimental and computational capabilities. The scientific disco...
July 21, 2015Chapter 9 — Enabling Capabilities for Science and Energy
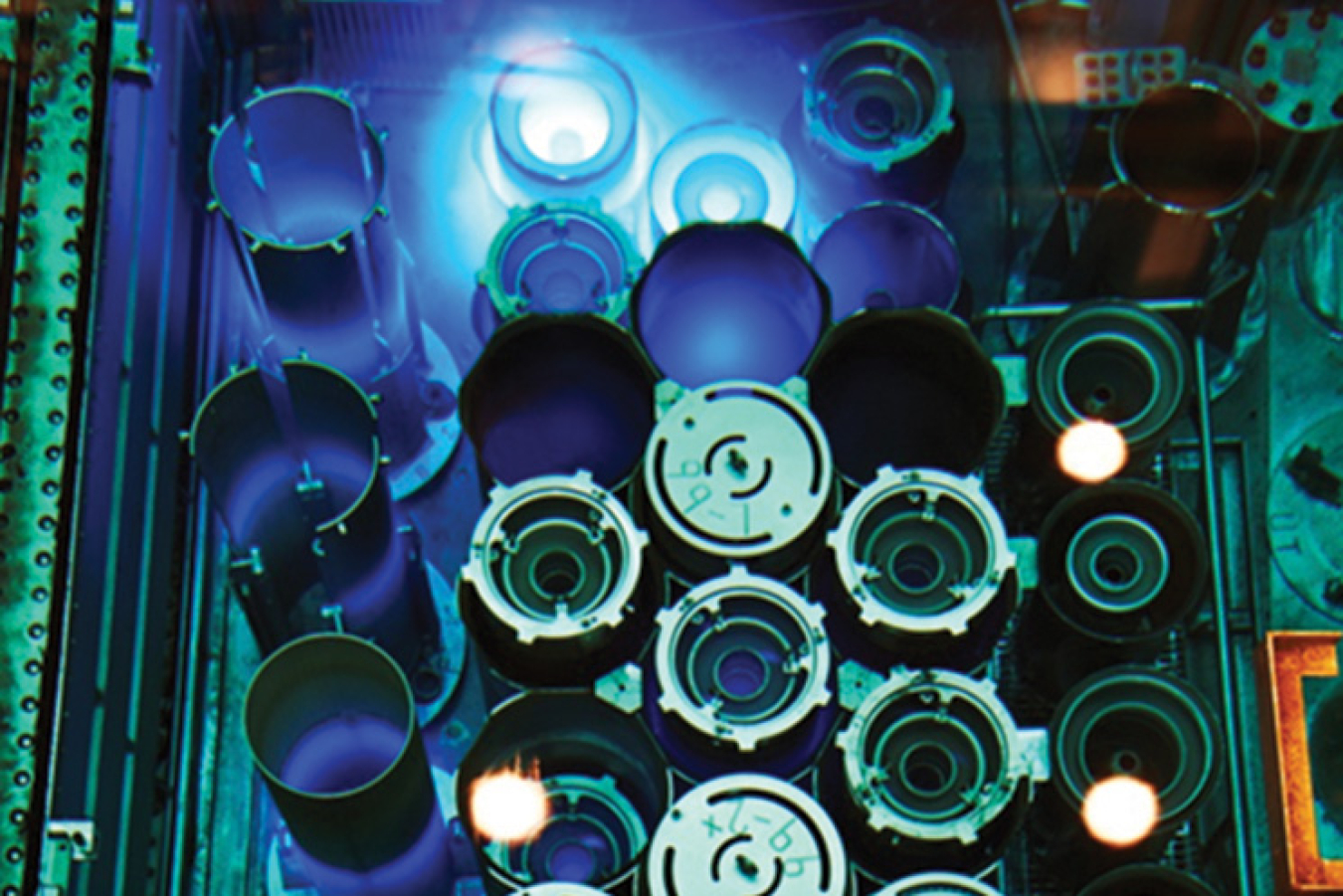
Basic science expands our understanding of the natural world and forms the foundation for future technology. Energy systems that meet our energy security, economic, and environmental objectives require a new generation of materials that may not be naturally available. However, creating these new materials requires a level of understanding of the relationships between structure and function, and across many spatial scales, which is not yet supported by our understanding of the physical world. Basic scientific research is necessary to fill these knowledge gaps and enable creation of new materials with the specific characteristics needed for next-generation energy technology.
In materials science, the current challenge is to understand how nanoscale phenomena translate to properties at the mesoscale and beyond. As systems grow in size from the nanoscale to the mesoscale, properties emerge that could be manipulated to produce desired functionalities in the bulk material. In this way, nanoscale design can result in the creation of radically new materials, with properties and functionalities that expand upon, or fundamentally differ from, those found in nature.
Analogous to inorganic materials, living systems demonstrate properties and functionalities that go beyond the additive functions of their constituent parts. The challenge for systems biology is to understand how particular changes to metabolic pathways—often stemming from small changes at the genome scale—play out at the level of the whole organism or an entire microbial community.
This new energy research agenda is being shaped by dramatic advances in computation. Today’s high-performance computers allow complex real-world phenomena to be studied virtually, including phenomena at the nano- and mesoscale, at very high spatial and temporal fidelity and at a much-accelerated pace. Critically, these tools are giving access to the properties of systems too dangerous to study experimentally, or too costly to develop by trial-and-error.
Taken together, these developments have put science and technology on the threshold of a transformation from observation to control and design of new systems. This paradigm shift is transforming the processes by which new materials and bio-systems are predicted, designed, and created. This revolution represents a convergence of theory, modeling, synthesis, and characterization, and will enable predictive modeling of materials, control of chemistry, and synthetic biology.
This chapter is a survey of how the Department of Energy (DOE) and the Office of Science support energy technology through investment in basic science research and development of complex and unique experimental and computational capabilities. Planning for and development of the capabilities described in this chapter is rooted in both the opportunities presented by basic science and the enabling tools needed by the research community to make the discoveries. The scientific discoveries and capabilities described in this chapter are also critically important for technology development, enabling discoveries that can obviate the technical roadblocks to broader implementation.
The chapter describes a flexible framework that can incorporate some or all of the methods listed above. This framework would be suitable for analyzing the impact of a RDD&D portfolio under a complex set of objectives and requirements.
July 21, 2015Chapter 10 — Concepts in Integrated Analysis
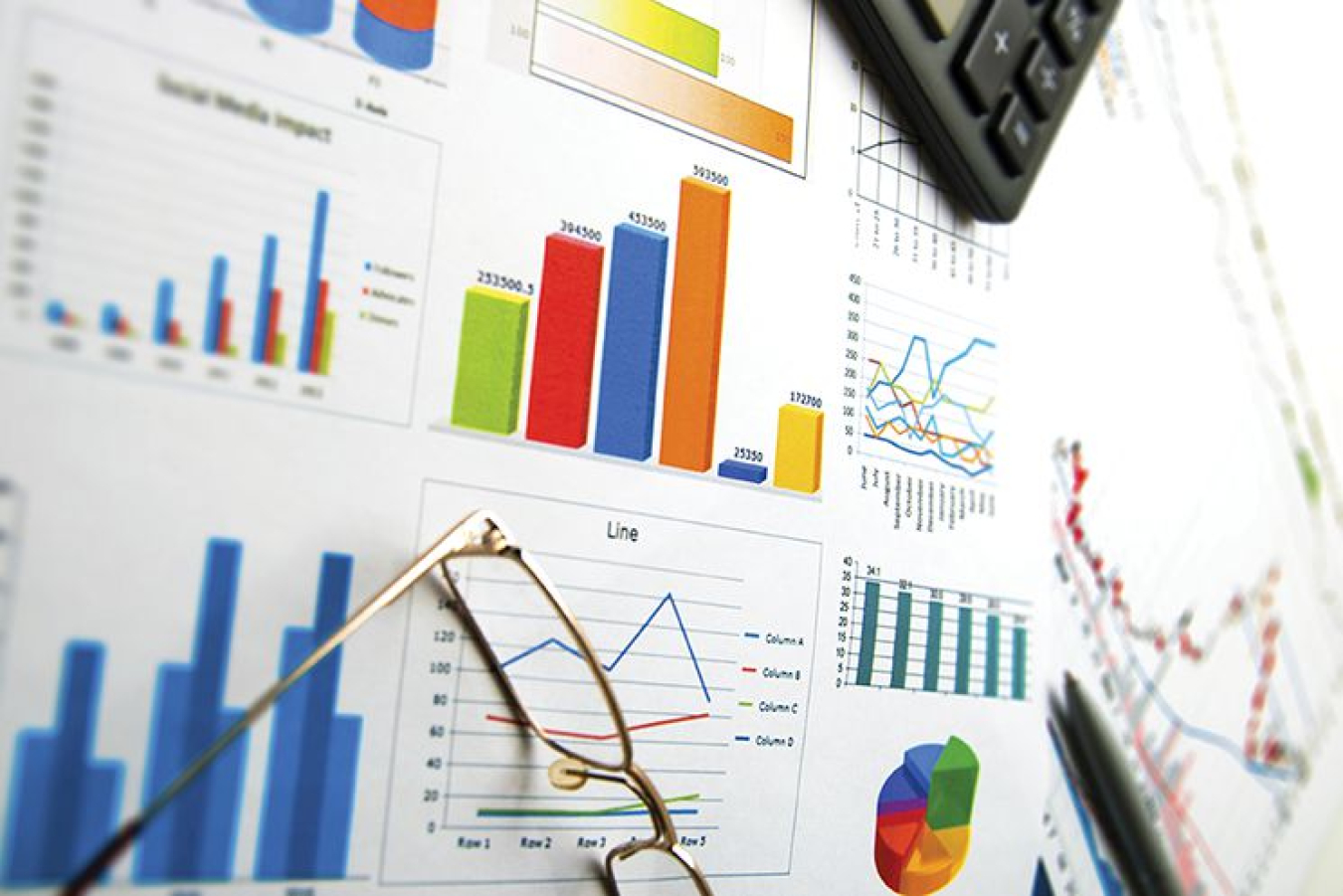
The goal of energy technology development programs, whether in the private sector or in government institutions, is to maximize the positive impact of research, development, demonstration, and deployment (RDD&D) portfolio investments. To evaluate total impacts, research institutions must consider multiple impact metrics that address energy-linked economic, security, and environmental goals from business and public perspectives. Portfolio analysis is widely employed, but at varying levels of thoroughness, analytic rigor and transparency. Many tools for technology planning and projection, analysis, metrics calculation, and impact evaluation exist already, but are not necessarily fully developed or packaged in a way that can be used directly for evaluating energy portfolios. This chapter does the following:
- Provides a suggested, iterative process to shape an energy portfolio and estimate the potential impacts of particular RDD&D activities on key national goals
- Articulates the current state of integrated technology assessment
- Gives examples of sector-specific applications of metrics and tools for technology analysis in use in various organizational contexts (i.e., corporate, nonprofit, academic, and government)
- Identifies gaps in technology assessment capabilities
Summary and conclusions of the 2015 Quadrennial Technology Review.
July 21, 2015Chapter 11 — Summary and Conclusions
To meet our nation’s strategic energy objectives of a secure, competitive, and environmentally responsible energy system, broad deployment of a range of advanced energy technologies will be needed. By approaching these reviews from an energy system perspective, four overarching themes emerged:
- Energy systems convergence
- Diversification within the energy sectors
- Energy efficiency everywhere
- Confluence of computational and empirical capabilities
Six sets of core opportunities for specific RDD&D activities are presented, organized by the energy sectors represented in the technical chapters of this report:
- Enabling modernization of electric power systems
- Advancing clean electric power technologies
- Increasing efficiency of building systems and technologies
- Innovating clean energy technologies in advanced manufacturing
- Advancing systems and technologies to produce cleaner fuels
- Advancing clean transportation and vehicle systems and technologies
Twelve cross-cutting technology areas are identified. These avenues of research with benefits across multiple sectors could be pursued through new research initiatives and/or by facilitating more communication between, and enhanced alignment of, existing activities. These cross-cutting technology areas are:
- Electric grid modernization
- Systems integration
- Cybersecurity
- Energy-water nexus
- Subsurface science and technologies
- Materials
- Fuel/engine co-optimization
- Energy storage
- Computational modeling and simulation
- Data and analysis
- Analysis of complex systems
- Characterization and control of material at multi-scales
These issues are summarized in this chapter.
<p><a href="/node/728131">Office of the Under Secretary for Science and Energy</a></p><p><a href="/node/1142411">QTR 2015 Overview</a></p>