Solar energy projects are one of the most popular forms of Tribal clean energy development and are a great place to get started.
Tribal solar projects can range from electrifying individual homes, to offsetting energy costs on large commercial or municipal buildings, up to developing "utility scale" solar arrays that generate energy equivalent to traditional power plants.
Explore the topics below to decide which solar options might best support your Tribe's energy goals:
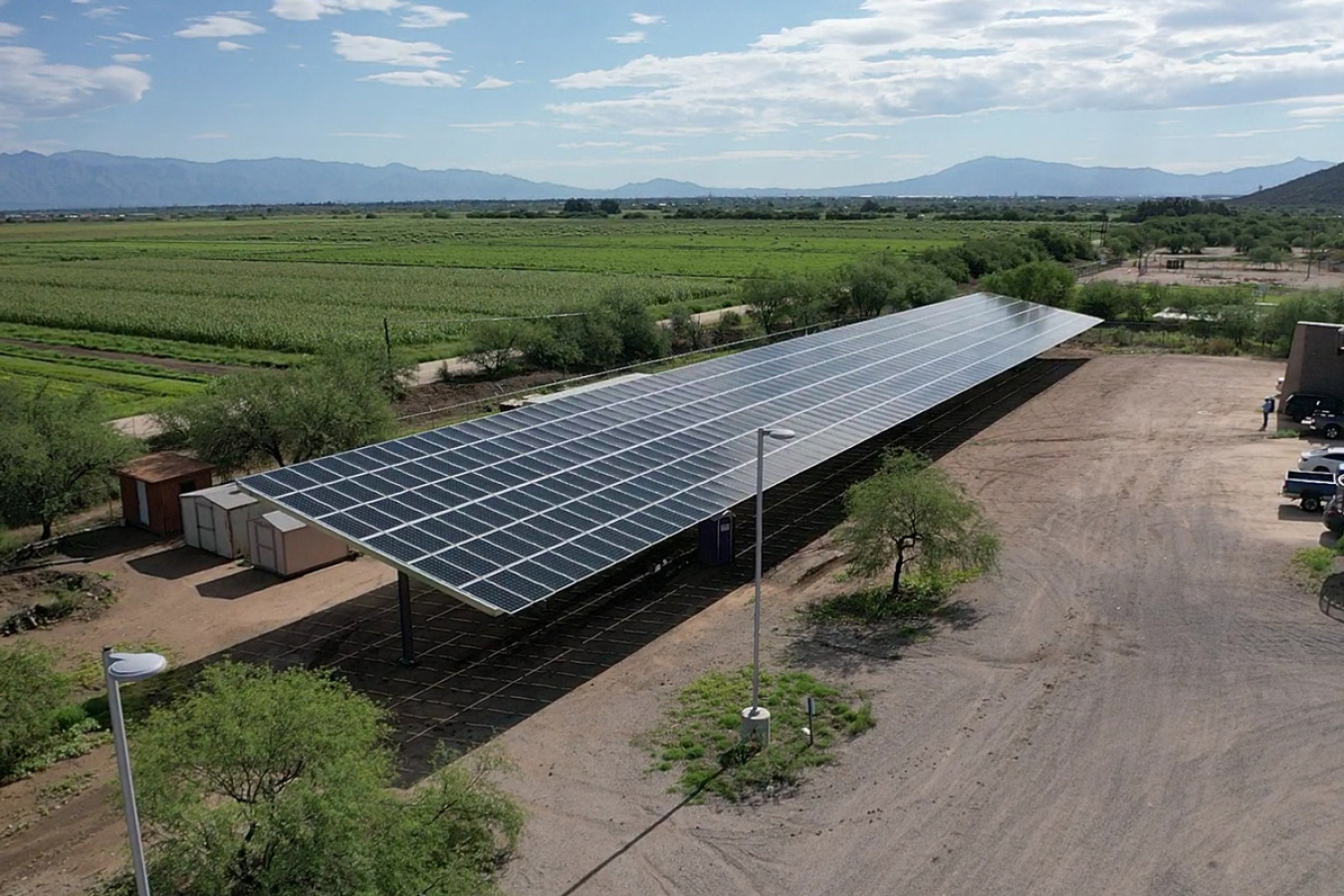
Solar Energy Resource
The solar energy resource is the sunlight that hits the earth’s surface. This light is known as solar radiation, which is captured and converted using technologies such as photovoltaic (PV) and solar thermal panels. Solar energy can be used to produce heat and electricity. While abundant, sunlight availability at the earth’s surface varies based on several factors including location, time of day, season, landscape, and weather. For more information, visit EERE’s How Does Solar Work webpage.
Components of Solar Radiation
The instantaneous solar resource is known as irradiance (power/area) and the amount of sunlight that is captured over a given time period is known as insolation or irradiation (energy/area). The solar resource hitting a surface has the following components:
- Direct Normal Irradiance (DNI): the solar radiation hitting a surface directly from the sun.
- Diffuse Horizontal Irradiance (DHI): the solar radiation hitting a surface that does not arrive on a direct path from the sun but has been scattered by molecules and particles in the atmosphere and comes equally from all directions.
- Global Horizontal Irradiance (GHI): the total amount of solar radiation received by a horizontal surface.
- Reflected Irradiance: the solar radiation received by a surface that has been reflected (typically) from the ground.
For more information, visit EERE’s Solar Radiation Basics webpage.
Solar Radiation Data Resources
Solar resource atlases typically provide the global radiation either for a horizontal surface (GHI) or for a surface oriented towards the equator and tilted at latitude (this orientation and tilt generally provides maximum annual insolation for a non-tracking surface). Read the fine print to ensure clarity on what is being presented.
- Tribal Energy Atlas (TEA): The TEA is sponsored by the Office of Indian Energy and it collects multiple types of Tribal energy data and information. The purpose of the TEA is to equip Tribes with the information and data needed to conduct their own analysis and understand their energy options.
- National Solar Radiation Database (NSRDB): The NSRDB provides data for three most common measurements of solar radiation: Global Horizontal (GHI), Direct Normal (DNI), and Diffuse Horizontal (DHI), as well as meteorological data.
- RE Data Explorer: Site housing renewable energy data and analytical tools to inform energy planning and decision making around the world.
Compared to other renewable energy resources, there is much less variation in the solar resource based on location. Within the continental United States, the solar resource varies by less than a factor of three and changes only gradually with distance.
Effect of Azimuth and Tilt on Collected Solar Radiation
The amount of solar radiation that is received and captured by a surface is highly dependent on the orientation (azimuth & tilt) of the surface.
The figure below shows the effects of tilt and tracking strategies on the total insolation received by a surface.
- As shown, a surface tilted at an angle equal to the latitude receives more solar radiation than a horizontal surface. Tilting the surface to an angle equal to the latitude also reduces the seasonal variation in insolation received by the surface.
- For fixed tilt arrays, orienting the panels towards the equator and tilting them at an angle equal to the latitude typically maximizes annual energy production.
- A tilt angle less than latitude will increase summer energy production while a tilt angle greater than latitude will increase winter energy production.
- As shown in the figure the annual energy production can also be further increased by tracking.
Solar Energy Technology
To make use of the energy provided by the sun, various technologies have been developed to absorb solar radiation and convert it to usable heat or electric energy. This section will discuss the most commonly known technology, photovoltaics (PV), which converts sunlight directly into electricity. For additional information about solar energy, visit:
Overview
The design of a PV solar system that will supply the desired amount of electrical energy to a building or community requires extensive planning and analysis. To do this, it is important to understand the components that are required to build a cost-effective, durable, and environmentally friendly system.
Solar pieces range in size from tiny cells that power calculators to residential/facility sized systems to utility sized power plants. The figures below show typical configurations for facility scale and utility solar systems. This section will elaborate on the mechanisms that produce a successful and long-lasting solar energy project.
Facility scale solar system configuration:
Utility scale solar system configuration:
To learn more, visit EERE’s Solar Photovoltaic System Design Basics webpage.
Solar System Components
Modules
PV modules convert sunlight directly into DC electricity. When sunlight is absorbed by the material in a module it transfers its energy to negatively charged electrons, which are knocked loose and flow through the full power system to produce electricity. The process of converting light (photons) into electricity (voltage) is called the photovoltaic effect.
Modules can be fabricated out of a variety of materials. The most common approach is to fabricate modules from individual silicon cells. PV cells are small and individually produce only 1 - 2 watts of power. To produce larger amounts of electricity individual cells are wired together, as shown below, to create a module with the desired nominal voltage and current. The other main approach to module fabrication is thin film technology. Being solid state devices with no moving parts, PV panels are very reliable with a typical warrantee period of 20 years or more.
Modules can be wired together to create an array of arbitrary capacity to accommodate the desired energy need.
Module capacity is described in terms of Wattsdc. This is the power produced by the panel under an irradiance level of 1.0 kW/m2 (roughly the level of sunlight at midday on a clear summer day). The array capacity is the sum of the individual module capacities.
Photovoltaic (PV) materials and devices convert sunlight into electrical energy.Department of EnergyTo learn more, please see the following webpages:
Mounting and Racking
Solar panels must be mounted on a structure that is sufficiently durable and stable to support the panels, keep the panels oriented as desired, and able to withstand harsh elements. Today, rack mounting is the most common method due to its simplicity of construction & installation, and its versatility.
A wide variety of racking systems exist depending on the specific situation. The main categories include:
- Roof mounted (non-flat) which is primarily used in residential and small commercial systems.
- Flat roof mounted is primarily used in commercial systems.
- Ground mounted systems are primarily used in commercial scale, community scale, and utility scale systems. Ground mounted systems may be fixed tilt or use 1-axis tracking. Small (commercial) PV systems tend to use fixed tilt mounting. Larger, utility size arrays, mostly use 1-axis tracking. Due to its propensity for failure, two-axis tracking is rarely used.
Inverter
PV systems that are grid connected or serve altering current (AC) loads require one or more inverters to convert the DC electricity generated by the panels into the AC electricity for used by most electric devices. There are three general arrangements of inverters, each offering different advantages and disadvantages:
- Microinverters are used at the module level. Each module has its own inverter. Microinverters eliminate the need for DC wiring but due to their higher cost are used primarily in residential and small commercial systems.
- Central inverters serve a whole array. This is often the least cost option. Disadvantages include the need for extensive (and expensive) DC wiring and reduced resiliency, the failure of the inverter will take the whole PV system off-line.
- String inverters are a compromise between microinverters and central inverters. Each string inverter serves a portion of the array. The failure of one inverter will only take a portion of the PV system off-line.
Another important inverter characteristic is grid following versus grid forming. Grid-following inverters only operate when connected to a functioning grid and shut off when grid power is unavailable. Grid forming inverters can operate when the grid is not available. If a PV system is to serve off-grid loads or used for back-up power, ensure that the inverter is grid forming.
Transformer
This component steps up the inverter output voltage to the grid voltage. It is mostly found in larger systems.
Disconnects
These are switches that allow for safe isolation of all or parts of the system.
Storage
Batteries allow for the storage of PV generated energy both at the residential and at the utility-scale level and allow use of PV generated electricity regardless of when it was produced. To learn more about energy storage, visit EERE’s Solar Energy and Storage Basics webpage.
Photovoltaic systems can be configured in numerous ways, ranging from simple to complex, depending on their use. PV systems can produce either direct-current (DC) electricity just like a battery or, with an inverter, can convert that DC current into alternating current (AC), as is used in most households. Within the U.S., AC grid-connected systems are the most prevalent.
DC Direct-Drive PV Configuration
This configurate is used to power off-grid DC loads that can use variable power and achieve their purpose while operating only part of the time, such as water pumping for cattle or irrigation. In this application the PV array is connected directly to a pump, which feeds a water tank or trough. A simple controller can disconnect the PV array when the tank is full. Due to the specialized nature of the loads which it can serve this configuration is uncommon.
DC PV Systems with Battery Storage
This system is used for DC loads that require a steady power supply or on-demand power. Lighting applications are a good example—these systems are often used for flashing warning lights. The systems require a controller to govern the flow of electricity to and from the batteries while maintaining a steady flow of power to the application. Note that using energy-efficient lighting will greatly reduce the needed size, and thus the cost, of the PV system.
Small individual DC systems have many applications, such as providing power for home systems, public area lighting, schools, health clinics, water pumping & purification, and rural telephony & micro-enterprise development.
Off-Grid AC PV Systems
This configuration is used for PV systems intended to power off-grid AC loads or for grid connected PV systems that provide backup power during outages. A typical off-grid AC PV system includes the PV modules, a bank of batteries to store PV energy for later use, a controller, and an inverter to convert DC power to AC power. This configuration allows the use a standard AC wiring and AC appliances.
Utility-Connected AC PV Systems
Depending upon the scale this configuration is used for PV systems intended to supplant utility power, or provide power to the grid. PV systems with this configuration can be used to supplement the grid during the day while the grid meets the building's power needs at night. At times when the PV system produces more power than is needed the excess power can be fed back into the power grid.
PV design tools are available to assist in sizing and configuring of envisioned PV systems; estimating the power output; and performing an economic analysis. Visit the following resources to learn more:
- PVWatts Calculator is an easy-to-use tool to estimate PV project technical and financial performance.
- System Advisor Model (SAM) allows for more detailed performance and financial modeling of renewable energy systems (including PV).
Rooftop
The array density for rooftop systems is roughly 15 Wattsdc per square foot of applicable roof surface. Note that it is unlikely that every square inch of south or southish facing roof surface can be used. A reasonable rule of thumb is to initially assume that roughly half to two-thirds of the applicable roof surface(s) can be covered with panels. For example, a south facing roof surface of 400 square feet could accommodate roughly 3,000 – 4,000 Watts (3.0 – 4.0 kW) of PV panel capacity.
Learn more by visiting EERE’s Solar Rooftop Potential webpage.
Ground Mounted
The density of ground mounted PV arrays is generally in the range 3-4 acres per MWdc. Note that this is the area actually covered by the arrays. A given PV project site will use additional land for space between the array and the fence, space between subarrays, unbuildable areas such as draws, etc.) For an arbitrary, reasonably flat parcel, a reasonable rule of thumb for the maximum PV capacity is roughly 5-6 acres per MWdc. Learn more by visiting:
Solar Energy Cost
Market Status
The rapid decline in PV costs in recent years has led to an explosive increase in PV deployment both worldwide and in the U.S. This growth is occurring at all project size levels from small distributed systems to large utility-scale systems. The figure below shows past and anticipated PV deployment in the United States. As can be seen, on a capacity basis, the utility-scale segment forms the bulk of current and anticipated deployment. 2021 capacity additions totaled 25,000 MWdc (25 GWdc).
Source: Utility-Scale Solar, 2022 Edition, http://utilityscalesolar.lbl.gov.
Capital Costs
The table below summarizes average unit capital costs of PV systems installed in 2021 as reported by Lawrence Berkely National Laboratory (LBNL). Note that these average values disguise a great deal of variability. This variability exists between different markets, within the same markets, and within size categories. In general, the larger the system, the lower the per-Watt cost. This is true even within broad size categories as demonstrated in the figure below. For non residential systems the average unit costs decline from $3.60/Wdc (for systems smaller than 10 kWdc) to $1.60/Wdc (for systems larger than 1,000 kWdc.)
Average Unit Cost for PV Systems Installed in 2021:
Market Segment
Capacity Range (kWdc)
Average Unit Cost ($/Wdc)
Residential
Up to ~15 kWdc
$3.80
Small Non-Residential (Commercial)
Up to 100 kWdc
$3.00
Large Non-Residential (Commercial)
100 – 5,000 kWdc
$2.00
Utility
> 5,000 kWdc
$1.02
Sources: Tracking the Sun (2022 Ed.), Utility-Scale Solar (2022 Ed.)
Average unit cost for non-residential PV systems installed in 2021. Source: Tracking the Sun.
Operating and Maintenance (O&M) Costs
O&M costs are less well defined in the literature and subject to greater uncertainty. One complication is that the definition of “O&M” is not consistent. The NREL Annual Technology Baseline (ATB) estimates annual O&M at about $30/kWdc for residential systems, and $20/kWdc for commercial and residential systems.
Lawrence Berkely National Laboratory PV Market Reports: These reports, sponsored by the United States Department of Energy and updated annually, give a good overview of cost, deployment, performance, and technology trends.
- Tracking the Sun (Systems up to 5 MW): https://emp.lbl.gov/tracking-the-sun
- Utility-Scale Solar (Systems larger than 5 MW): https://emp.lbl.gov/utility-scale-solar/
Lazard’s Levelized Cost of Energy Analysis: This document, updated every 1-2 years, gives high level cost ranges and economic performance analysis for various energy technologies, including PV.
Solar Energy Industries Association (SIEA): A trade association representing the solar industry, the SEIA website has resources, (a portion of which are available for free), such a quarterly and annual market reports, pertaining to PV deployment, market status, and issues.
American Clean Power Association (ACPA): A trade association representing the wind, solar, and battery industries, the ACPA website has resources, (a portion of which are available for free), such a quarterly and annual market reports, pertaining to PV deployment, market status, and issues.
Featured Projects
Ute Mountain Ute Tribe – 2017 Project
Bishop Paiute Tribe – 2016 Project