Researchers study the energy and angular dependence of how neutrons scatter off materials to improve reactor safety and efficiency.
October 27, 2022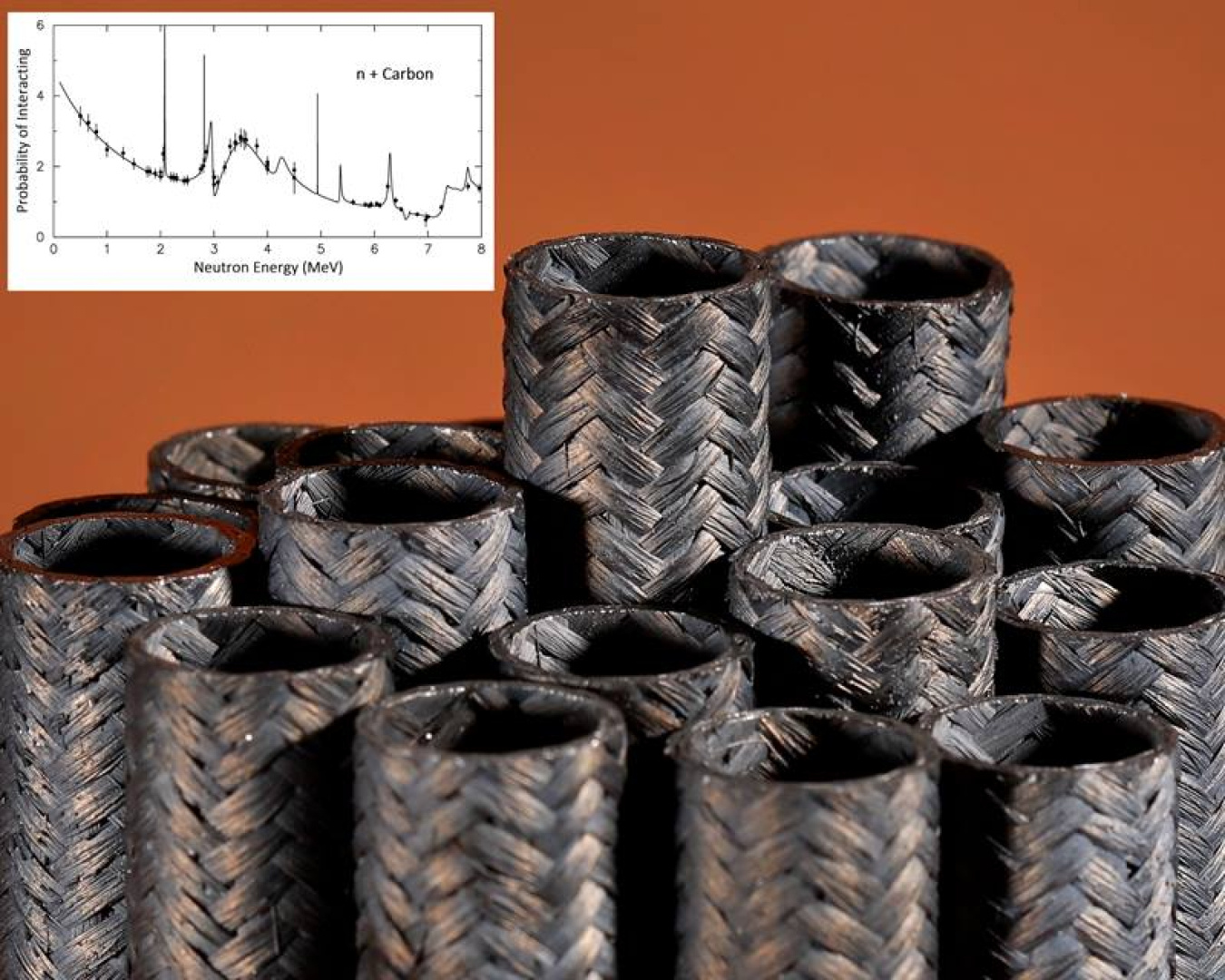
The Science
Nuclear fission and fusion reactors use the elements carbon and silicon as shielding and structural materials. Nuclear engineers also use these elements in fuel and in neutron moderators, which control the speed of neutrons to help maintain chain reactions. Silicon carbide, for example, can be used to clad fuel and as a pellet coating that offers protection from accidents such as the one at the Fukushima Daiichi power plant. Neutrons are the drivers of the nuclear energy production processes. This makes understanding how neutrons scatter from all reactor materials very important. In this research, scientists investigated the interaction of neutrons with silicon and carbon.
The Impact
Neutrons scatter from nuclei in ways similar to how balls scatter in a billiards game, except the scattering is ruled by quantum mechanics, as well as by conservation of energy and momentum. Researchers need a detailed knowledge of exactly how these uncharged particles interact with materials because these nuclear data are embedded in modeling and simulation software. These data also determine how well a system will perform. In this research, scientists measured the energy and angle dependence of neutrons scattering from silicon and carbon. The results provide answers that scientists can only uncover through experiments.
Summary
Many applications rely on global theoretical models of how neutrons interact with nuclei over a wide range of incident neutron energies. These applications range from energy production to homeland security to medical treatments. Scientists develop these models by comparing calculations with experimental data. Excellent agreement between data and theory indicates that the interaction between the neutron and the material is well understood. This process helps scientists gain confidence in their understanding of the nuclear force, and it helps engineers develop safer and more efficient reactors and scanners.
Carbon and silicon are just two such important materials investigated at the University of Kentucky Accelerator Laboratory by a collaboration of scientists and students from the University of Kentucky, the United States Naval Academy, Mississippi State University, and the University of Dallas. The energy (e.g., see inset image) and angular dependence of neutrons scattering from these materials revealed regions where scientists can improve theoretical models, especially for excited states in each nucleus, which in turn provides improved models for neutron behavior for many diverse applications.
Contact
Sally F. Hicks
University of Kentucky and University of Dallas
sally.hicks@uky.edu
Steven W. Yates
University of Kentucky
yates@uky.edu
Funding
This research was supported by the Department of Energy (DOE) Office of Science, Office of Nuclear Physics, the DOE National Nuclear Security Administration Stewardship Science Academic Program, and the DOE Nuclear Energy Universities Program. Additional support was provided by the National Science Foundation and the Donald A. Cowan Physics Fund at the University of Dallas; isotopes used in this research were supplied by the U.S. Department of Energy Isotope Program.
Publications
Ramirez, A. P. D., et al., Neutron elastic and inelastic scattering differential cross sections on carbon. Nuclear Physics A 1023, 122446 (2022). [DOI: 10.1016/j.nuclphysa.2022.122446]
Ramirez, A. P. D. et al., Neutron elastic and inelastic scattering cross section measurements on silicon from 0.8 to 8 MeV. Nuclear Physics A 1024, 122474 (2022). [DOI: 10.1016/j.nuclphysa.2022.122474]