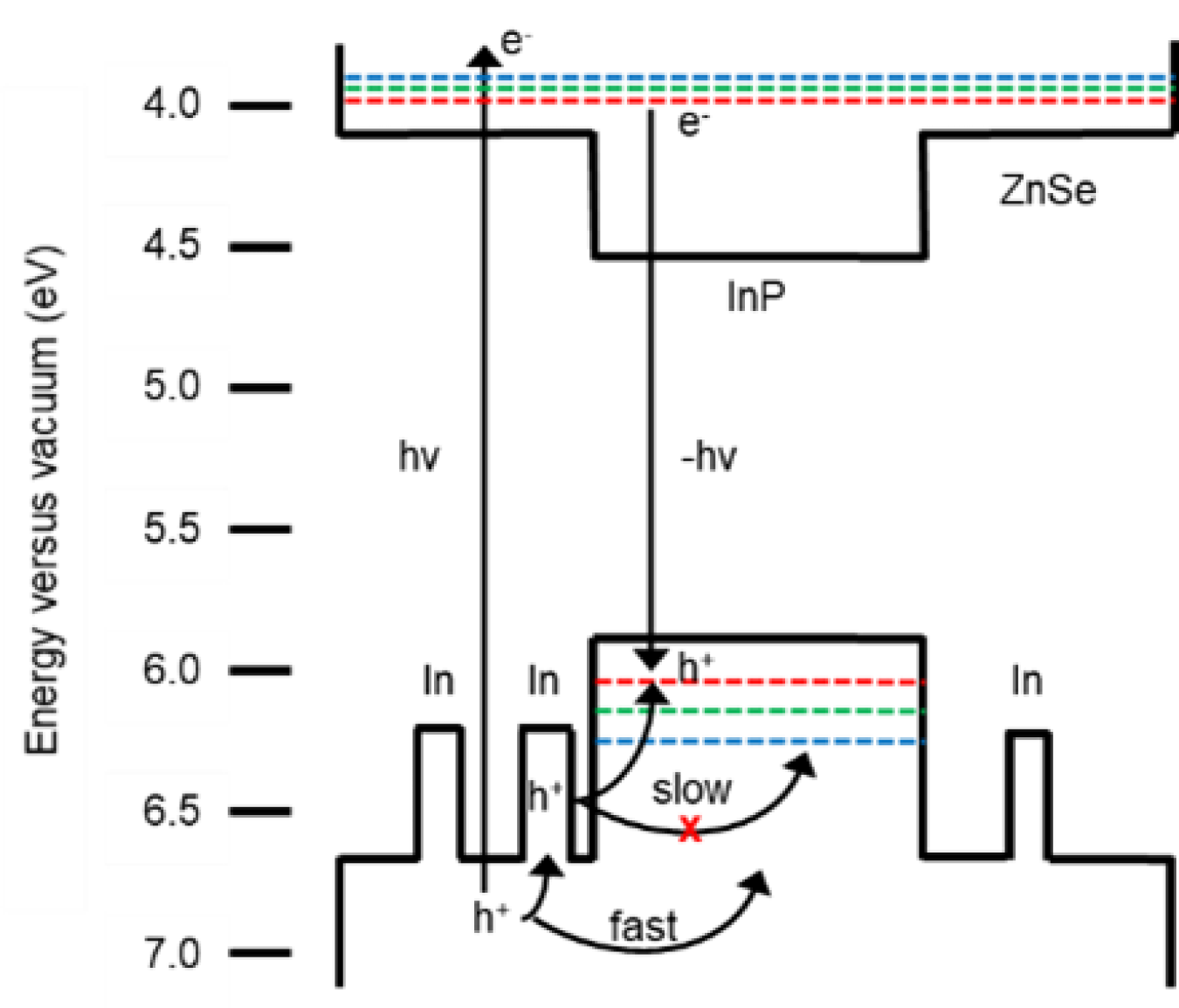
Down-converting emitters on LEDs absorb higher energy light, typically blue or near-ultraviolet, and re-emit the light at lower-energy wavelengths in a combination that produces white light. Quantum dots (QDs) are efficient emitters that have the potential to significantly improve the efficiency of LEDs, but two problems stand in the way: most QDs contain cadmium, which is a toxic and regulated material; in addition, QDs as emitters must operate in an environment with high light flux levels of 1 W/mm2 and temperatures up to 150°C, which can limit their performance.
Researchers at Nanosys, in partnership with the University of California-Merced, are working to characterize state-of-the-art, heavy-metal-free QDs from structural and photophysical perspectives. They seek to use this information to determine the best approaches to improve QD materials under LED operating conditions of high light flux and temperature. Project priorities include achieving structural attributes that improve these performance parameters and increase lifetime under these conditions. The goal for lifetime increase is 100-fold, with an ultimate project goal to create QD materials that do not contain toxic elements and operate on an LED without degradation for the lifetime of that LED. Research efforts focused on three activities: synthetic control of the QD structure, characterization of those structural changes, and stress testing under high-temperature and high-flux operating conditions to find the correlation between the QD photophysics and resulting performance. These activities have enabled researchers to gauge the performance status of current state-of-the-art, cadmium-free QDs to understand the underlying degradation mechanisms that lead to loss of emission power while controlling QD composition to improve operational lifetime.
Recent progress includes extensive photophysical characterization of novel InP-based core/shell QDs, which shows that carrier trapping rates increase with a greater In/P ratio (> 1:1), causing an additional step in the pathway to QD luminescence. The resulting model showed that absorption of blue excitation light by the ZnSe shell layer can result in temporary carrier trapping of holes on the excess indium sites within the ZnSe, causing a delay between excitation and emission. Research revealed that this temporary trapping phenomenon can affect the behavior of multiexciton states and leads to an increase in the likelihood of Auger electron excitation relative to Auger hole excitation.
From conducting these experiments on InP-based QDs with controlled concentrations of non-stoichiometric indium, researchers found that across the concentration range studied, the Auger electron excitation fraction more than doubles from 40 to 85%. Critically, a correlation was observed between higher electron-excitation fractions and a corresponding increase in the high-flux operational stability of the studied QDs. This information has allowed researchers to modify the core/shell structure of the InP-based QDs for the highest operational stability to date, lasting to a T70 lifetime (70% retention of initial emission power) of 4,000 hours when tested at 50°C and 50 mW/mm2 — representing a 50X improvement since the project start.
Future work will focus on understanding the chemical nature and energetics of the indium-associated trap states to yield further stability improvements for cadmium-free QDs under high-flux excitation. (August 2021)
Return to Research Highlights.