Microelectronics projects will support more powerful supercomputing, explore new materials, foster advanced computing architectures, and more.
August 26, 2021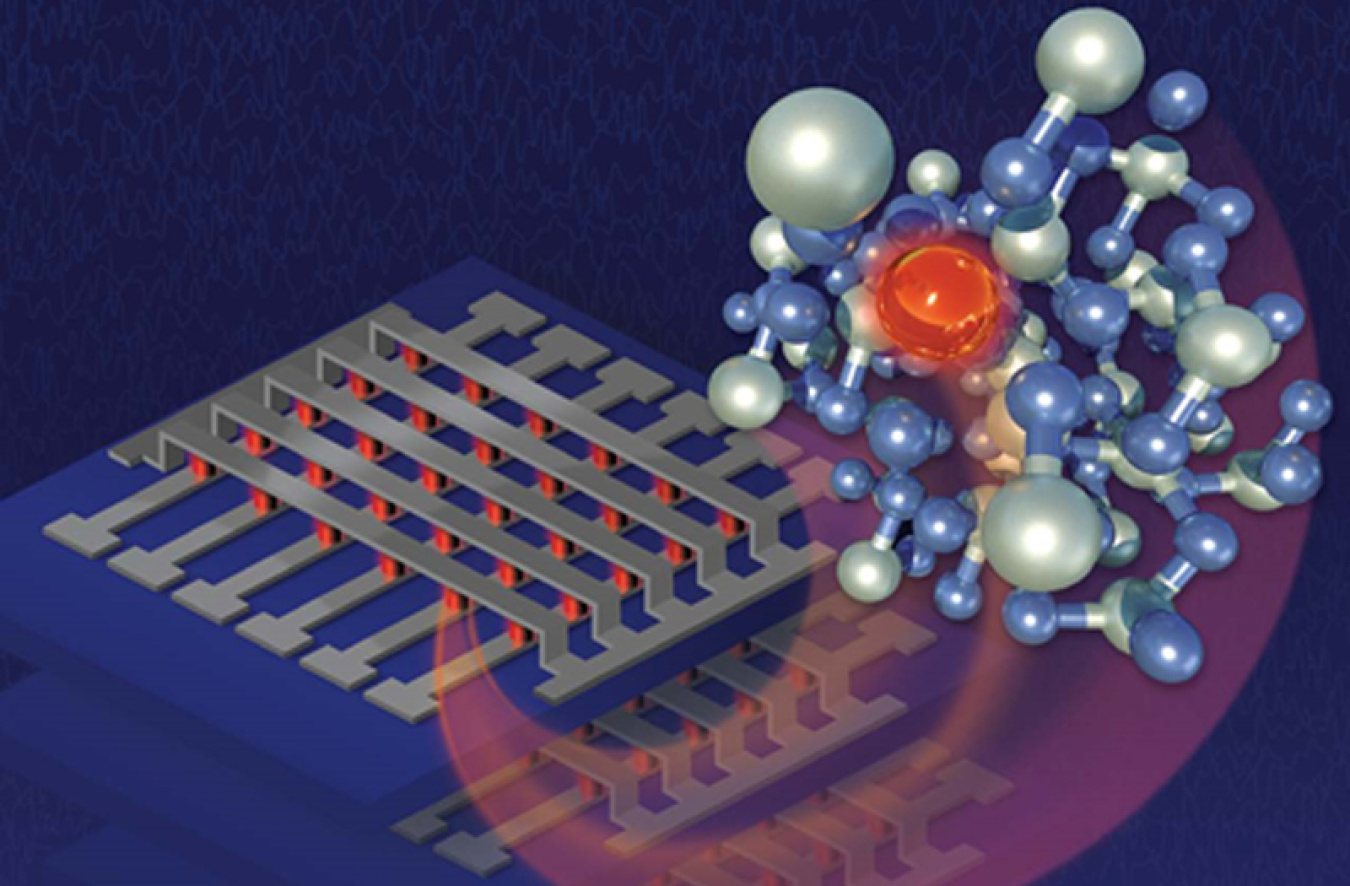
Microelectronic devices are vital to nearly every aspect of our lives—from running a small business to driving the global economy, from tracking our personal health to fighting a pandemic, and from delivering power to our homes to securing our nation’s infrastructure.
Microelectronics include computer chips, power electronics like those that control electricity, and other small semiconductor devices. Since the mid-20th century, microelectronic devices have rapidly decreased in size and cost and increased in performance and energy efficiency—changing the world in a short time. However, these transformative devices are now facing technical and economic challenges that will require new innovations. This second revolution in microelectronics will apply new understandings in the physical and computational sciences.
For decades, the U.S. Department of Energy Office of Science and the DOE national laboratories have worked with U.S. industry to develop and demonstrate scientific advancements for microelectronics. The Office of Science also operates many scientific user facilities open to the research community that depend on a range of microelectronic devices, including sensitive particle detectors, sophisticated microscopes, intense X-ray and neutron sources, as well as data centers, networks, and high-performance computers.
That’s why the Office of Science created a microelectronics initiative with the goal of stimulating U.S.-based innovation as the foundation for future domestic technology development and manufacturing. Office of Science-funded microelectronics projects will support ever more powerful supercomputing capabilities, explore new materials and fabrication methods, foster advanced computing architectures, and stimulate research and development for a range of microelectronics important to the DOE missions of scientific discovery, energy efficiency, and national security.
Since the 1970s, the semiconductor industry has routinely shrunk the size of transistors, from micrometers to nanometers. The smartphone in your pocket is more powerful than supercomputers were half a century ago—thanks, in part, to miniaturization. Every 18 months or so, chip manufacturers packed in twice as many transistors—famously known as Moore’s law. But as transistors approach the size of atoms, Moore’s law for classical computing is breaking down due to the complex laws of quantum mechanics.
The challenge extends beyond transistor size. Most computer processors are based on the 70-year-old von Neumann model, named for its inventor. In this model, the processing unit is separate but connected to a memory unit, which requires instructions from the processing unit and data from the memory unit to be shuffled back and forth during computation.
This extended data movement consumes energy and creates heat—the so-called von Neumann bottleneck. For supercomputing and data centers, this means building costly power and cooling infrastructures. For scientists wanting to analyze large amounts of data in real time during experiments, memory access and capacity and other data bottlenecks are frustrating roadblocks to scientific discovery.
To confront challenges like the end of Moore’s law and the von Neumann bottleneck, the Office of Science is funding research to design new materials that take advantage of properties at the atomic and subatomic scale. We are also funding research to develop new computing models, including neuromorphic computing that mimics how the brain works and quantum computing that leverages the physics of quantum mechanics to solve new types of complex problems. Data-driven software programs in artificial intelligence and quantum information science will benefit from these new architectures designed for their unique purposes. And there may be others—we will likely see a combination of or hybrid computing models in the future.
However, it’s not just about computers. As the United States upgrades its 100-year-old electricity grid, microelectronics will be the key to adding more renewable energy sources, protecting against cyberattacks, and introducing a two-way flow of electricity between the consumer and the grid, which will optimize use. Scientists are also planning for how future microelectronics will improve research. Energy-efficient, data-nimble microelectronics will allow researchers to collect and analyze more data faster, using devices closer to experimental setups.
The key to accomplishing all this requires a collaborative “co-design” approach that brings together experts from the beginning to the end of the microelectronics pipeline. Historically, each step in the R&D process was carried out independently. However, through co-design, the Office of Science will leverage materials and chemical scientists, mathematicians, computer engineers, industry partners, and others to work together to inform each step of the process and innovate better and faster.
Knowing the big impact that microelectronics has on our lives today, it is critical to prepare this important technology for the future. Microelectronics projects led by the national laboratories will help us bridge the gap between the discovery science we need to understand how to advance microelectronics and introducing these new technologies into the lab and the marketplace.
The Office of Science is the single largest supporter of basic research in the physical sciences in the United States and is working to address some of the most pressing challenges of our time. For more information, please visit www.energy.gov/science.
Katie Jones
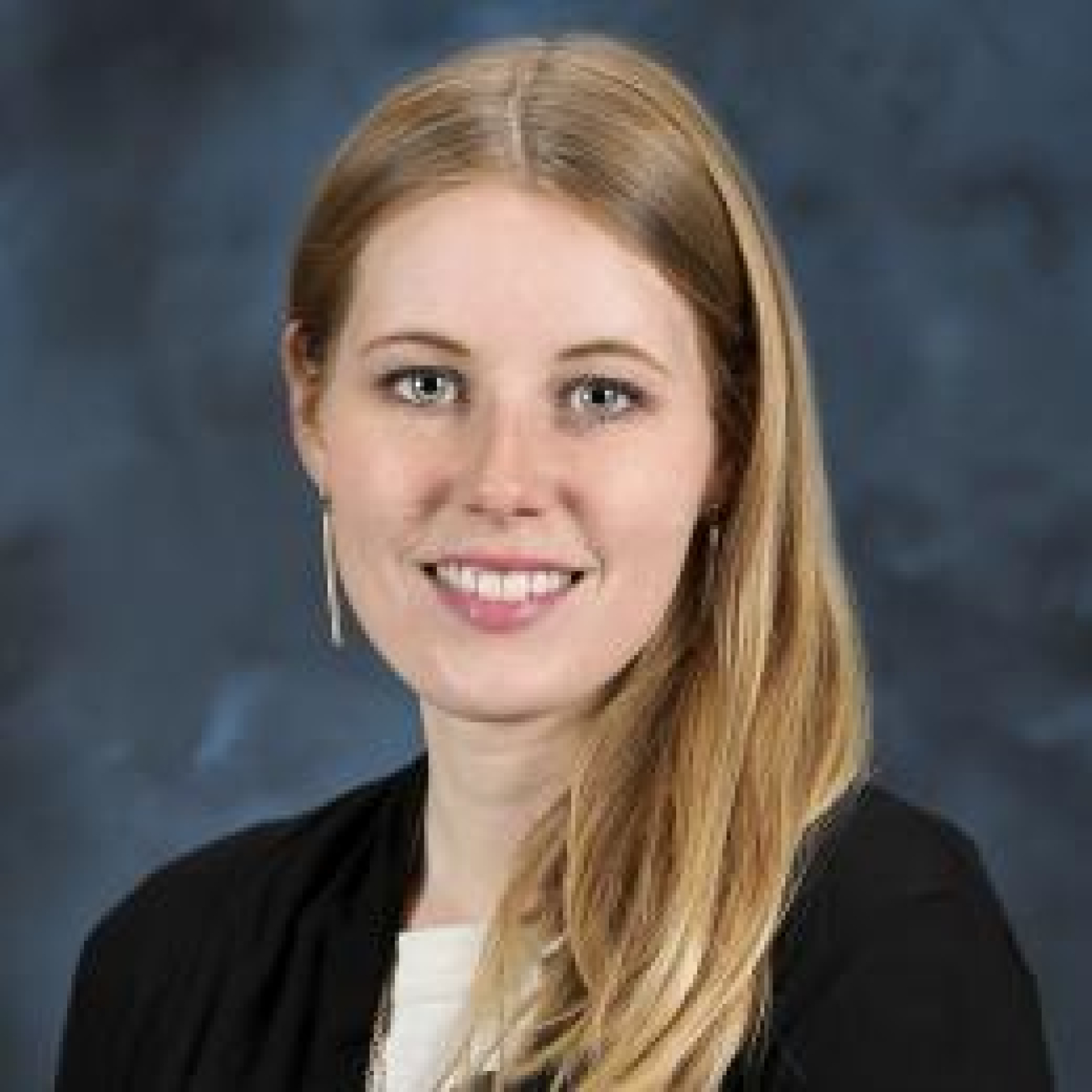
Katie Elyce Jones was a science writer for the Office of Science in the Office of Communications and Public Affairs, on detail from Oak Ridge National Laboratory. She wrote feature articles, video scripts, and other content. Katie has over a decade of experience writing and editing about science and energy research at several Department of Energy national laboratories. She has covered topics such as supercomputing, particle physics, energy technologies, and climate science. Katie holds a bachelor’s degree in journalism and science communication and a master’s degree in English literature and creative writing.