Scientists and engineers are in the process of building and testing the infrastructure for the Deep Underground Neutrino Experiment.
October 13, 2023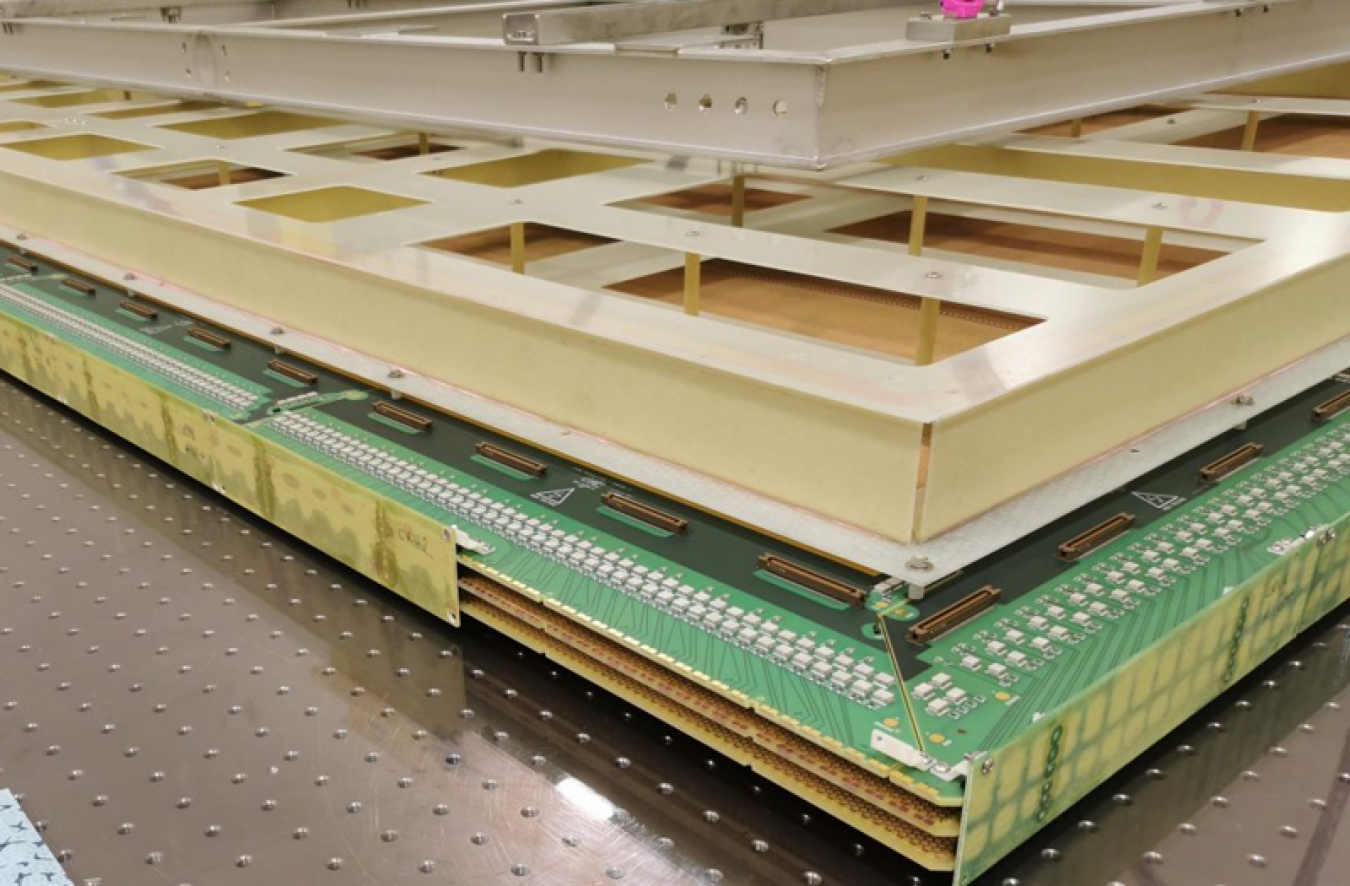
If there’s something strange in the universe, who you gonna call? Particle physicists!
That response may not be as catchy as “Ghostbusters!” but there is actually a “ghost particle” haunting our world. Neutrinos are the most common matter particle in the universe. Trillions of them move through our bodies every second without ever interacting with us. They could hold the key to explaining why matter dominates the universe instead of antimatter or unify the theories of how the four major forces of the universe work. Unfortunately, neutrinos hardly ever interact with anything. That makes them incredibly difficult to study.
To better understand this strange little particle, scientists and engineers are in the process of constructing the Deep Underground Neutrino Experiment (DUNE) and its infrastructure at the Long-Baseline Neutrino Facility. The experiment relies on both a particle accelerator at the Department of Energy’s Fermilab and a huge particle detector in South Dakota. The particle accelerator will produce a powerful neutrino beam that will travel from Fermilab in Illinois 800 miles (1,300 kilometers) through the Earth to reach the far detector in South Dakota.
Fermilab is the host laboratory for the project, but it involves scientists from all over the world. Physicists at the CERN particle physics center in Switzerland are testing the components of two of the detector modules.
The detectors use two different types of technology but are based on the same principles. Both are filled with liquid argon. Argon is a gas at room temperature and is a normal part of Earth’s atmosphere. Because neutrinos interact with so little, the scientists need to maximize the number of possible interactions. Hence the argon is cooled to very low temperatures to liquify the gas and increase the number of atoms in the detector modules. When a neutrino does interact with an atom of argon, it produces new particles. As the particles move through the argon, they knock out electrons. The detector has a strong electric field that pushes the electrons along, like a teacher herding small children into a classroom. The electrons eventually reach the particle detector systems that gather data. By measuring when and where the electrons hit the structure, scientists can develop illustrations of the particles’ paths. In addition, when a neutrino interacts with an argon atom, it also creates a flash of light. Sensors inside the detector modules gather data on those flashes of light, which provides additional information.
Functionally, the main thing that distinguishes the two detectors is how the electrons are being detected. The first detector is a horizontal drift detector. In this one, the electrons move sideways. It’s based on an existing technology that engineers are scaling up to use in DUNE. The second detector is a vertical drift detector. In this one, the electrons move up or down. The technology for the vertical drift detector is new. It took a full two years of testing for scientists to determine that it was ready for prime time.
What makes the scientists interested in the vertical drift detector is that it uses an easier to manufacture method to detect and collect the electrons. The horizontal detectors use special, tightly wound wires. In contrast, the vertical detectors use circuit boards that are simpler, cheaper, and easier to install. In addition, they leave more room for the argon. Being able to have a larger volume of argon means that there’s the possibility for even more neutrino interactions. If this technology works as well or better than the existing one, this could lead to more detectors that use the new technology.
In addition, the newer detector has a bigger, more comprehensive, and more sensitive system to detect the flashes of light. That improvement makes it able to collect more data. It’s also more likely to be able to detect low-energy events that produce neutrinos, like from exploding stars called supernovae.
Once the team at CERN finishes and fully tests the prototype, they’ll move on to getting the components in place in South Dakota. Right now, they’re planning to have it ready to be installed by 2027.
“Ghostly” neutrinos may not cause any frights, but scientists are definitely interested in solving some of their mysteries.
Shannon Brescher Shea
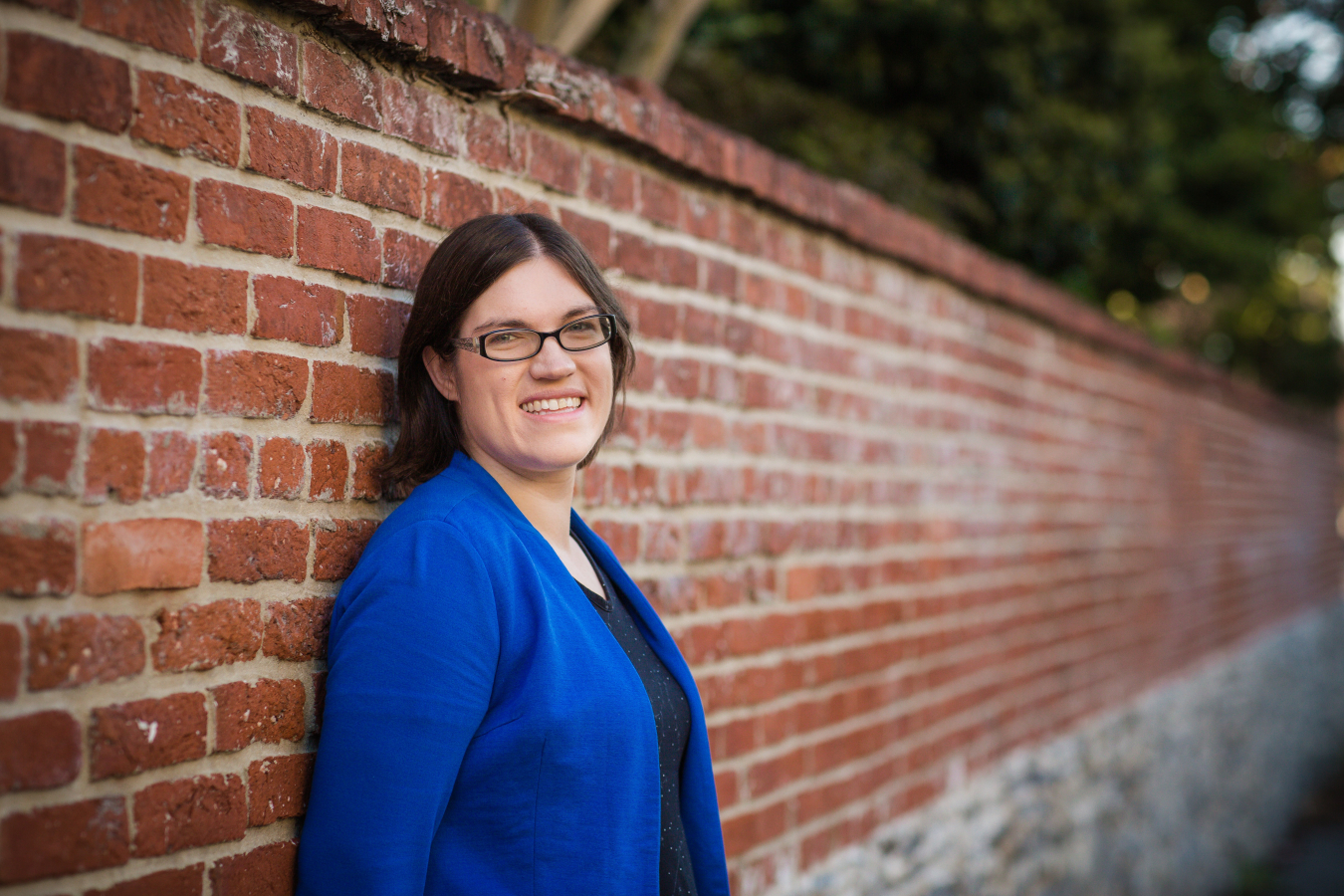
Shannon Brescher Shea (shannon.shea@science.doe.gov) is the social media manager and senior writer/editor in the Office of Science’s Office of Communications and Public Affairs. She writes and curates content for the Office of Science’s Twitter and LinkedIn accounts as well as contributes to the Department of Energy’s overall social media accounts. In addition, she writes and edits feature stories covering the Office of Science’s discovery research and manages the Science Public Outreach Community (SPOC). Previously, she was a communications specialist in the Vehicle Technologies Office in the Office of Energy Efficiency and Renewable Energy. She began at the Energy Department in 2008 as a Presidential Management Fellow. In her free time, she enjoys bicycling, gardening, writing, volunteering, and parenting two awesome kids.