How Nobel recipient W.E. Moerner and his team built the ABEL Trap and discovered the behavior of single, unfettered molecules.
October 5, 2016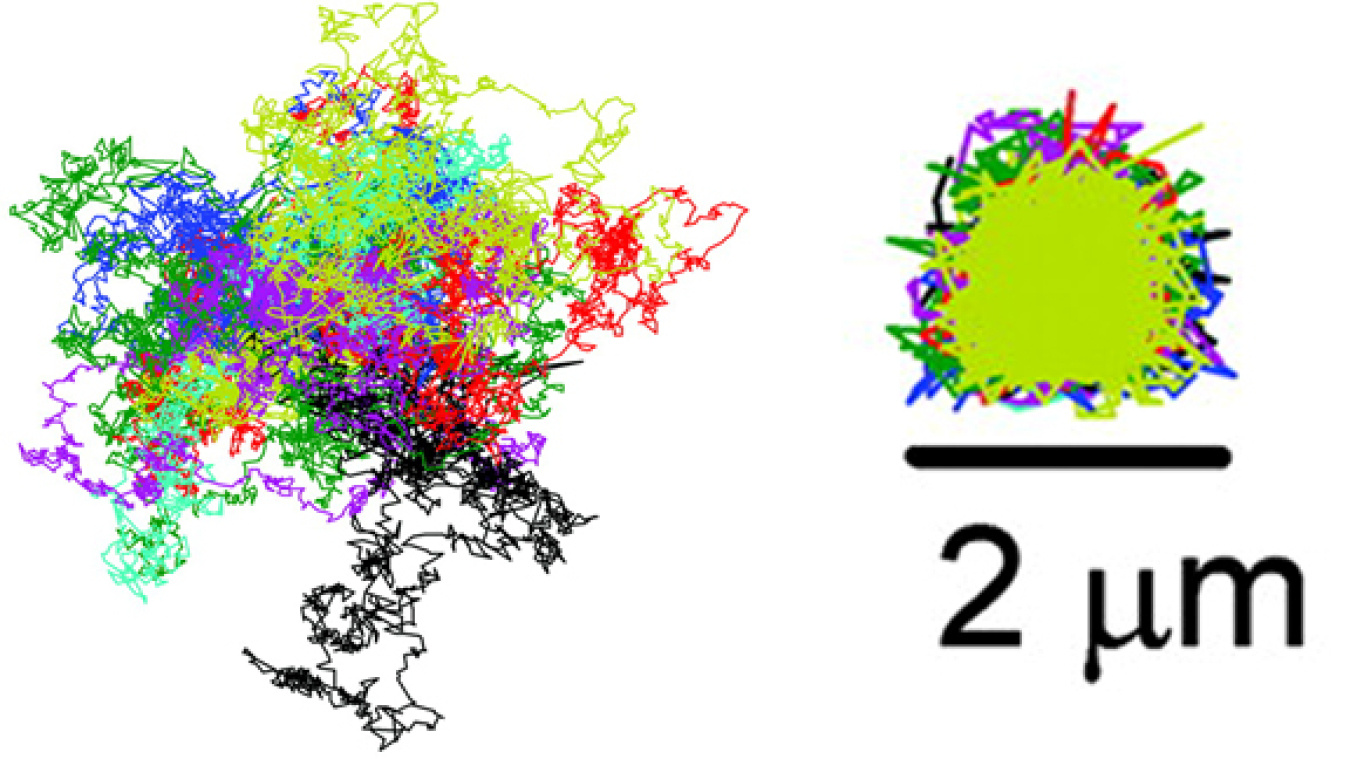
This graphic illustrates how the ABEL Trap can corral individual particles of tobacco mosaic virus. The graphic on the left shows the trajectories of 13 particles that are similar to the trajectories the particles would have followed if they had not been trapped. The graphic on the right is the trajectory of the trapped particles the researchers measured. The scientists trapped each particle for 6.8 seconds.
Water molecules are constantly jostling and kicking protein molecules about. Known as Brownian motion, this poses a challenge to scientists who want to study a single, unbound protein. Just like a baseball coach wants to know how the star player batted, scientists want to know how proteins perform alone.
Scientists typically overcome this challenge by artificially tethering the particles to a surface. However, tying down particles can change their behavior.
Enter Chemistry Nobel Prize recipient W.E. Moerner. He won the scientific community's highest honor for his imaging techniques that use single molecules to show the inner workings of cells on tiny scales. While some might rest on their laurels, Moerner hasn't. With his team at Stanford University, he has successfully taken on the challenge of corralling single molecules in water. By holding them in place, scientists can evaluate the performance of individual members of the team.
For example, inside every leaf, tiny solar-powered chemical factories produce sugary fuels and chemicals. Industries want processes that mimic such processes, pumping out chemicals without using rare metals, extreme heat or high concentrations. To do so means you have to know the details of the reactions inside nature's factories down to the behavior of single protein molecules. But until Moerner, there was no good way to study these proteins.
Moerner's work to defeat Brownian motion began back in 2004 and drew the interest of DOE Office of Science program manager Bob Stack; his program strongly contributes to Moerner's research. "In the beginning, it was high risk but also high reward," said Stack. "This is the kind of problem that is rarely solved over a single three-year grant cycle, which made it the kind of project our core programs are designed to support."
Corralling Molecules
Moerner and graduate student Adam Cohen built a device that corrals a single molecule. They named the device the Anti-Brownian ELectrokinetic Trap, or ABEL Trap. To use the trap, each protein, virus or other object of interest gets a fluorescent tag that shows the object's location. The device tracks the tag using a specialized camera and laser beams connected to a computer.
The computer calculates the particle's position in the solution to see how far the water molecules have kicked the tagged object.
The device then applies electric fields in two dimensions that effectively counteract the water's jostling, bringing the particle back into the field of view of the camera. The fields corral the particle in such a small space that it appears to be nearly standing still, making it easier to examine with a host of scientific tools.
Moerner and Cohen published the first paper on the ABEL Trap in 2005. In it, they describe how the device caught and held tiny polystyrene spheres, the compact, diminutive cousin of packing peanuts. The spheres were about 200 nanometers in diameter, or about the same distance across as a small bacterium.
"No one had done this before," said Moerner. "Nobody could trap single tiny objects in solution directly."
The Secrets of Photosynthesis
Moerner, along with the students in his lab, began applying the ABEL Trap to proteins involved in photosynthesis. They examined photosynthetic antenna proteins that capture light. These shuttle the energy from the light to a specialized complex that starts a series of reactions that use the energy to create the chemical bonds inside sugars and other molecules.
Moerner and postdoctoral fellow Randall Goldsmith repeatedly trapped an antenna protein called allophycocyanin. They found that a single protein gives off light far more subtly than just on or off – the light varies in intensity in fascinating steps. "You can't see this looking at the bigger picture of many molecules," said Moerner. "You can only see it by being able to study one molecule at a time."
In another study, Moerner and his colleagues used the ABEL Trap to catch similar single proteins, but this time each protein held several different pigments. They examined how the pigments moved and reorganized in response to bursts of light. They revealed that two chemically identical pigments on the protein play vastly different roles. One pigment absorbs red light, bringing in more energy for the cell to use. The other pigment protects the protein from taking in too much energy and being damaged.
"The insights we are gaining into how nature works can inform the design of future energy systems that are sustainable and driven by the sun," said Stack.
The trap also offers insights into the behavior of single molecules that are not involved in energy production and storage. Scientists at other universities are using the device to delve into questions about human and crop health.
Build, Test, Study, Repeat
The team continually strives to improve the device with ongoing support from DOE's Office of Science. Recently, Moerner's postdoctoral fellow Quan Wang improved the device's ability to snag molecules that are just 1 nanometer in diameter, which is 100,000 times thinner than a sheet of paper. It can also hold the molecules for up to 10 seconds, which is an eternity in this type of research.
"Moerner and his colleagues have enabled us to carefully evaluate the performance of each member of the molecular team," said Stack. "His work with the ABEL Trap is providing exciting insights into how Mother Nature does the remarkable chemistry of photosynthesis and other biological processes."
The Office of Science is the single largest supporter of basic energy research in the physical sciences in the United States and is working to address some of the most pressing challenges of our time. For more information please visit http://science.energy.gov.
Kristin Manke is a Communications Specialist at Pacific Northwest National Laboratory on detail to the U.S. Department of Energy's Office of Science, kristin.manke@science.doe.gov.