Lithium-ion batteries (LiBs) are the ubiquitous power supplier in all consumer electronics, in all power tools and—as many companies and countries pursue greenhouse gas emission reduction goals...
April 6, 2021Background
Lithium-ion batteries (LiBs) are the ubiquitous power supplier in all consumer electronics, in all power tools and—as many companies and countries pursue greenhouse gas emission reduction goals—a growing proportion of the global light-duty automobile fleet. To work, these energy storage devices must have a place for the lithium ions to move to when the battery is working. This is the cathode, and it’s also the place that lithium ions come from when the battery is charged. In order to get enough energy from the batteries, LiB cathodes are made of various combinations of transition metals and oxygen in a particular arrangement. The best combination for many energy storage needs involves a cathode structure that is largely composed of cobalt (Co) ions. Even with the rise in cell phone use, this reliance on cobalt had not been a major hinderance, since only a small amount of Co was needed for these devices. Electrifying the worldwide automobile fleet with LiBs, however, changes the situation significantly.
Cobalt is considered the highest material supply chain risk for electric vehicles (EVs) in the short and medium term. EV batteries can have up to 20 kg of Co in each 100 kilowatt-hour (kWh) pack. Right now, Co can make up to 20% of the weight of the cathode in lithium ion EV batteries. There are economic, security, and societal drivers to reduce Co content. Cobalt is mined as a secondary material from mixed nickel (Ni) and copper ores. This means the supply is not independent of other commodity businesses and introducing new recovery projects is expensive. Moreover, the United States does not have large reserves for Co, and the extraction and early stage processing is concentrated in a small number of countries outside the United States. Therefore, the United States is looking to secure sources of Co, to drastically reduce the Co content in LiBs, or both.
Three different transition metals—Co, manganese (Mn), and Ni—can manage the brunt of the charge storage shifts, and many other metals including aluminum (Al), titanium (Ti), iron (Fe), and magnesium (Mg) help. However, simple cobalt oxide offers the best mix of providing a high voltage, yielding very good energy density, and moving Li+ ions around easily. Moving away from high Co content means the new cathode materials must be optimized for all of these performance characteristics via subtle changes in the arrangement of the transition metals and their relative compositions. Despite how good the transition metal oxide-based cathodes, usually abbreviated NMC for the three majority transition metals followed by the Ni/Mn/Co ratio, have become for EV batteries, there is a globally recognized need to reduce dependence on Co without sacrificing performance.
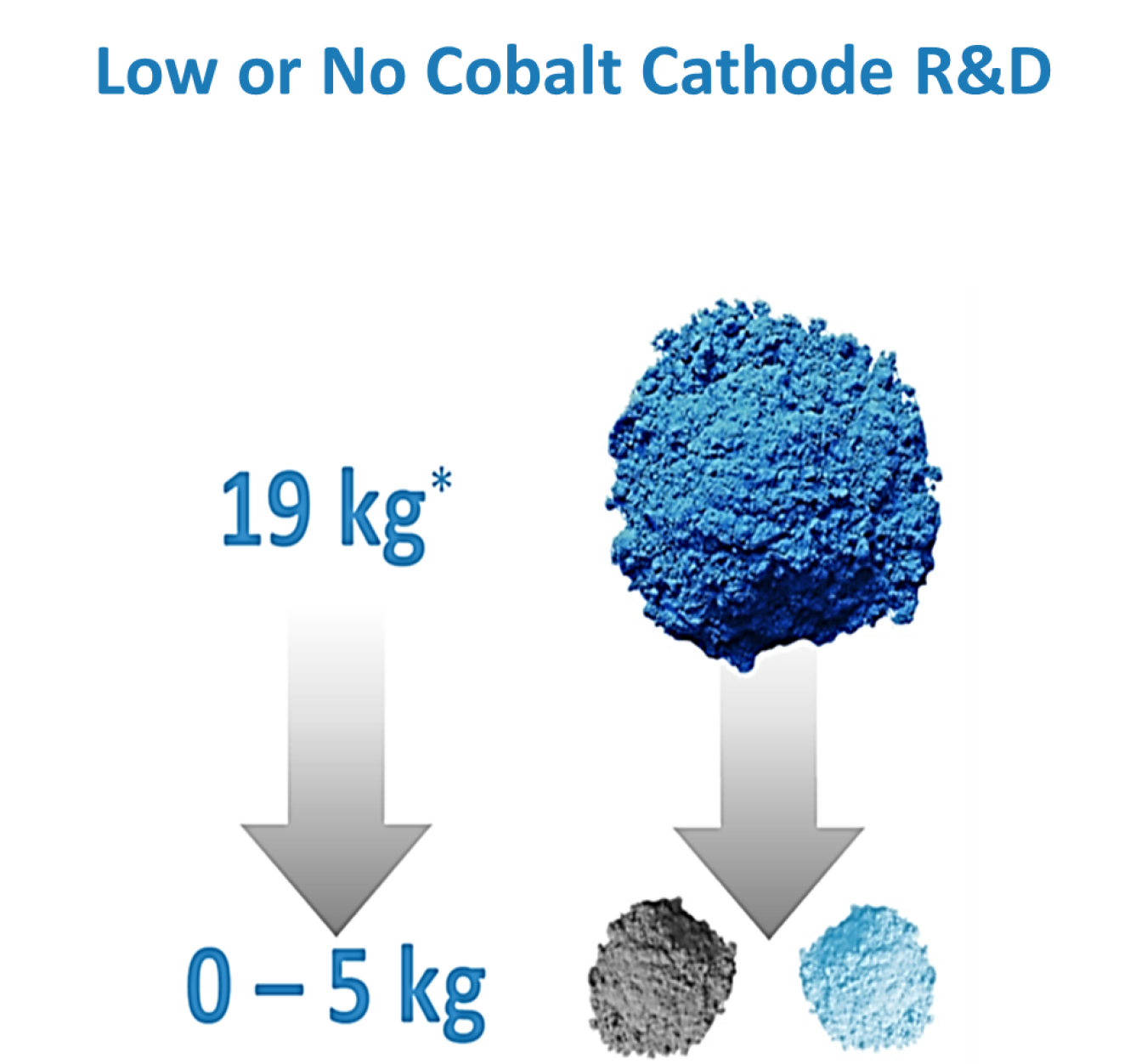
DOE R&D in Low/No Co Batteries
Industry has recognized the risks of Co dependency, and many battery manufacturers and end users have established ambitious goals to move to low- or no Co-containing cathodes. Many technological hurdles exist , and the U.S. Department of Energy (DOE), through the Vehicle Technologies Office, has committed to a multi-year, multi-thrust program to address all the scientific and engineering issues with eliminating most of the Co in EV batteries.
Many of the research projects are focused on high Ni materials, which exhibit very good energy density. To date, the challenge with using high Ni cathode materials is that they tend to suffer from rapid capacity fade and impedance rise due to deleterious reactions between the Ni atoms on the surface of the cathode and the cell’s electrolyte. Many projects are working to stabilize or protect that surface to minimize those reactions. The following highlights DOE-funded projects that have shown early success.
A research team led by Oak Ridge National Laboratory (ORNL), with contributions from XALT Energy and Nissan Technical Center, is developing a new class of Co-free cathode materials labeled NFA, composed of Ni, Fe, and Al. Figure 1 shows the cycling results for full cells made using the ORNL-NFA cathode material (LI1.0Ni0.85Fe0.052Al0.091O2). Most cells using NMC cathodes with Ni concentrations above 80% would experience much more rapid capacity fade than that shown here.
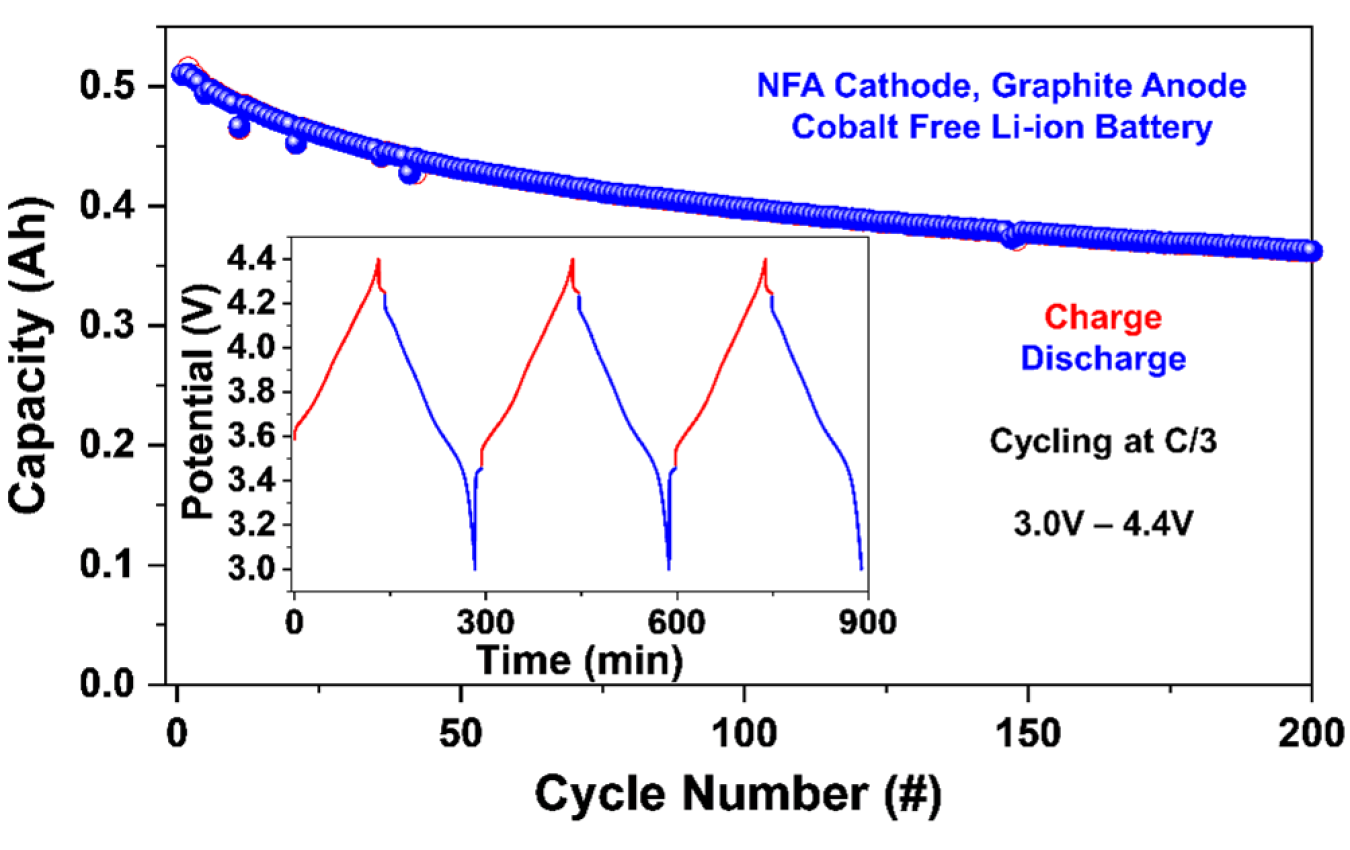
Figure 1. Cycling results for a Co-free Li-ion cell using NFA cathode.
A team led by the University of Texas at Austin with the National Renewable Energy Laboratory and Tesla Inc. is developing a range of cathode materials that incorporates low or no Co. Figure 2 depicts cycling results of several of these cathode materials in full pouch cells. The light blue line shows good cycling of commercial NMC622 (20% Co), which is exceeded or equaled by that of cells using NMA (90% Ni, 5% Mn, 5% Al, 0% Co) (grey line) and NMCAM materials (90% Ni, 4% Mn, 4% Co, 1% Al, and 1% Mg) (green line). The finding here is that cathode dopants (Al, Mg) can stabilize these materials at high-voltage cycling.
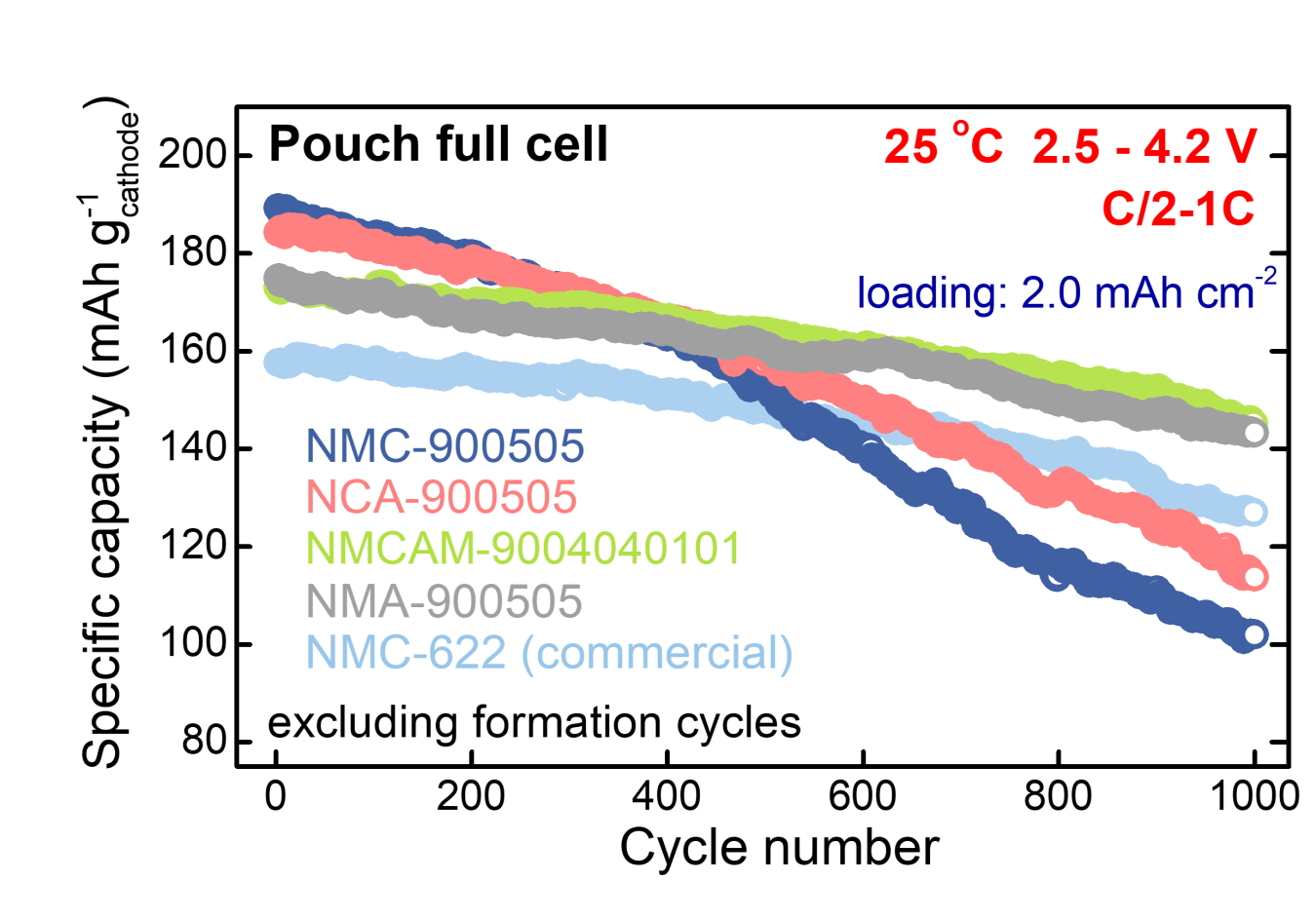
Figure 2. Full cell pouch cell cycling of low and no cobalt cathode materials.
A no Co cathode material synthesized using surface/bulk Ti/Mg doping, labeled NTM (LiNi0.96Ti0.02Mg0.02O2) has shown promising results in terms of cycle life and higher upper voltage limits (Figure 3). The team, led by the University of California, Irvine, and Virginia Tech—inspired by first-principles modeling results from Lawrence Berkeley National Laboratory with electrolyte R&D contributions from Pacific Northwest National Laboratory and full cell builds and testing from American Lithium Energy Corporation—continues to work on improve the stability of this high Ni cathode material.
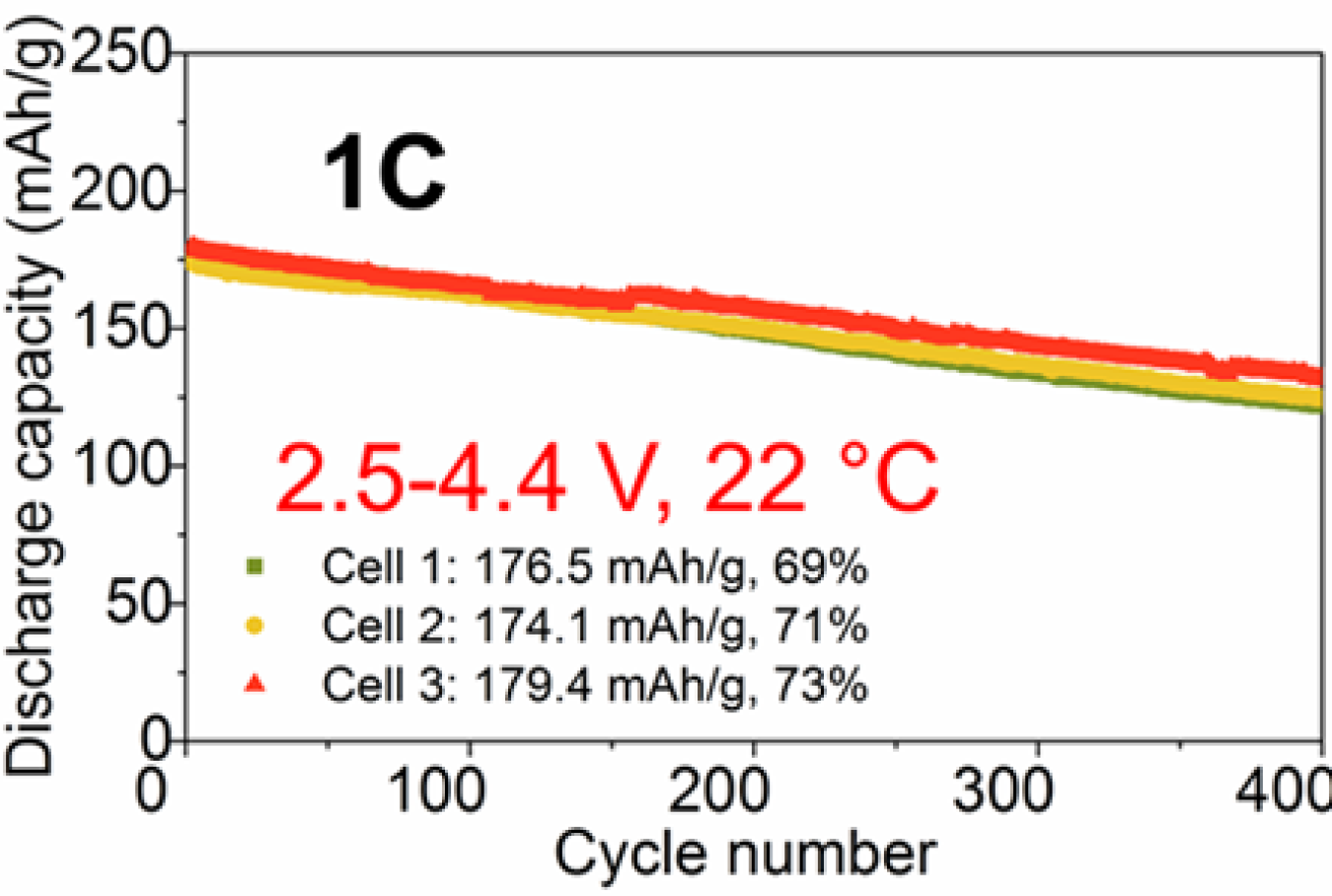
Figure 3. Electrochemical performance of the Co-free NTM cathode reaching a cycle life of 400 cycles (2.5 – 4.4V).