The Hydrogen and Fuel Cell Technologies Office's sorbent storage materials research focuses on increasing the dihydrogen binding energies and improving the hydrogen volumetric capacity by optimizing the material's pore size, pore volume, and surface area, as well as investigating effects of material densification.
The Hydrogen Storage Engineering Center of Excellence has developed a system projection graph showing a modeled MOF-5 system and how it compares against all of DOE's 2020 targets.
Download the final report for the Hydrogen Sorption Center of Excellence.
Technical Overview
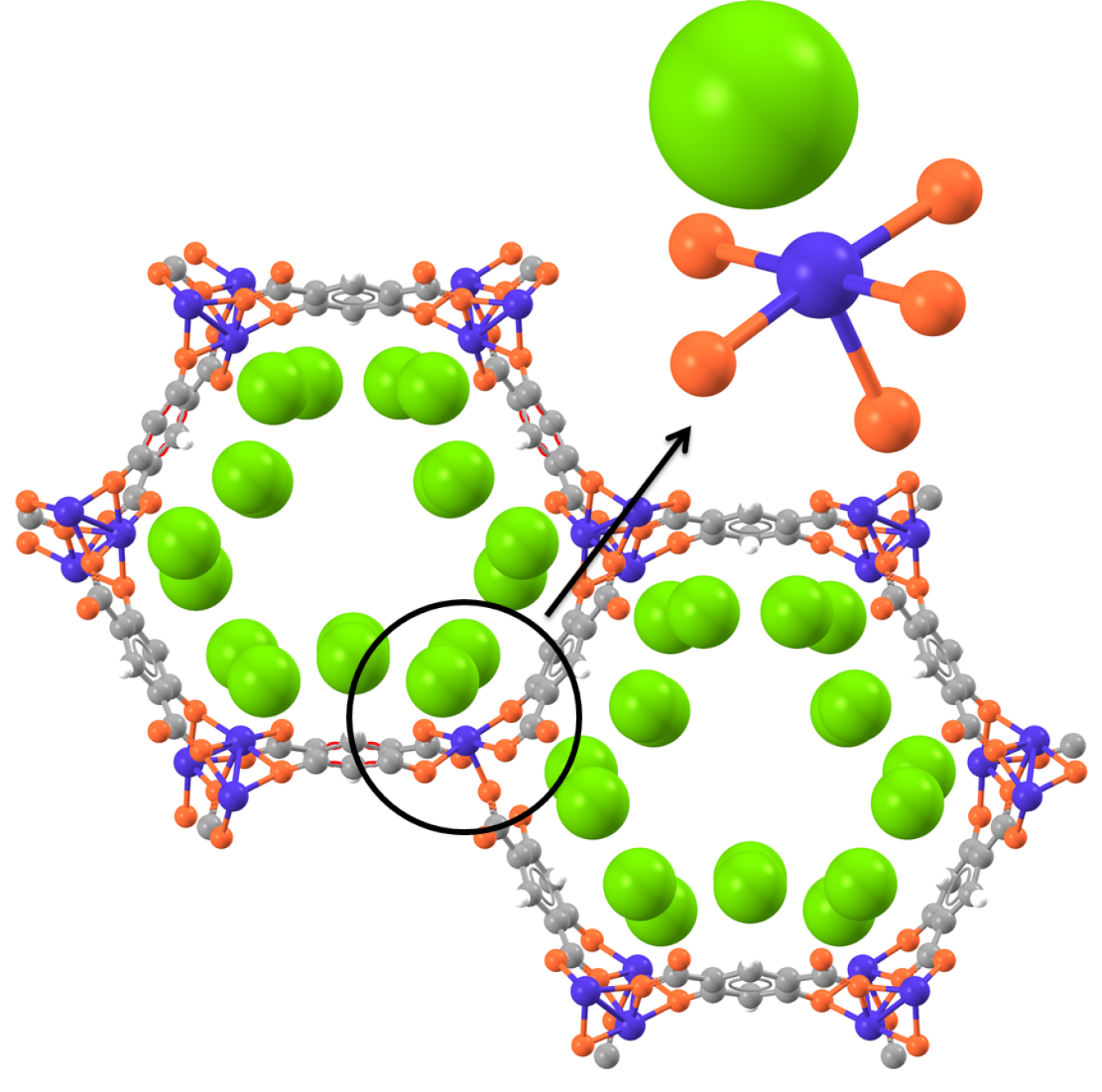
Unit cell of a coordinatively unsaturated metal center Co2(m-dobdc) structure dosed with three deuterium molecules (green) per open metal site. The upper right inset shows the location of the deuterium with respect to an open cobalt site.
Unlike other forms of solid-state storage, one of the advantages of using adsorbents as a storage medium is that dihydrogen retains its molecular form throughout the adsorption/desorption cycle with minimal activation energy. The primary disadvantage of using sorbents is the relatively weak adsorption enthalpies that are typical of gas-solid interactions when compared to that of bond formation with chemical hydrogen, or interstitial atomic hydrogen in metal hydrides. Additionally, the van der Waals dimension of molecular hydrogen is large in comparison to that of atomic hydrogen, putting limits on the overall volumetric density that systems based on dihydrogen can achieve.
Under many conditions, the nature of the adsorption process can still offer volumetric density advantages over the use of compressed gas storage,1 although not as high as intermetallic hydride densities. For dihydrogen that adsorbs onto a substrate, the London dispersion forces that can be regarded as transient dipoles of polarizable substances2 provide a convenient framework in appreciating the nature of adsorption above the triple point temperature and pressure.
Unlike chemical hydrogen or metal hydrides, the quantity that is measured either gravimetrically or volumetrically is the Gibbs surface excess.3 This quantity is a true measurement of increased concentration of gas at the surface of an adsorbent over the concentration that might be expected from the gas law and is the only value that is determined empirically.
The enthalpy range for hydrogen adsorbents can run typically from 5 to 10 kJ/mole. For sorption, the term "enthalpy" is not a single valued quantity, although it is often referenced this way. When reported, a single enthalpy value generally refers to the "differential enthalpy of adsorption at zero coverage," more commonly referred to as the Henry's Law value, where the gas concentration varies linearly with pressure.4 The Henry's Law value is essentially the enthalpy or potential assigned to the initial molecule adsorbed onto a surface. This value will generally be the highest quantity as the initial molecule adsorbs onto the highest potential site and adsorbs free of the influence of neighboring adsorbed gas molecules. Of more general technological interest is the isosteric enthalpy of adsorption, which traces the change in enthalpy over as large a range of coverage as pressure measurements allow. In all known instances for hydrogen sorption, the isosteric enthalpy of adsorption is expected to decay monotonically.
The sorbents that have been investigated recently range from coordination polymers5 to activated carbons. These classes of materials have the initial requirements for high gravimetric density as adsorption relies on having a high number of sites, upon which gas molecules can adsorb. For activated carbons, we can generally expect the absolute quantity of adsorbed gas molecules to vary as a function of surface area and micropore volume.6 As the gravimetric adsorption is highly correlated with surface area, maximizing surface area consistent with retaining a high micropore volume is the geometric combination that will best satisfy the design criteria for successful adsorbents.
References
- Chahine, R.; Bénard, P. "Adsorption Storage of Gaseous Hydrogen at Cryogenic Temperatures." In Advances in Cryogenic Engineering, Vol. 43. P. Kittel, ed. New York: Plenum Press, 1998; pp. 1257–1264.
- Parasegian, V.A. Van der Waals Forces. New York: Cambridge University Press, 2006.
- Gibbs, J.W. The Scientific Papers of J. Willard Gibbs. Woodbridge: Ox Bow Press, 1993 (reprint); p. 219.
- Rouquerol, F.; Rouquerol J.; Sing, K. Adsorption by Powders and Porous Solids. London: Academic Press, 1999.
- Robson, R. "Design and its limitations in the construction of bi- and poly-nuclear coordination complexes and coordination polymers (aka MOFs): a personal view." Dalton Transactions, 2008; pp. 5113–5131.
- Chahine, R.; Bose, T.K. "Characterization and Optimization of Adsorbents for Hydrogen Storage." Hydrogen Energy Progress XI: Proceedings of the 11th World Hydrogen Energy Conference. International Association for Hydrogen Energy, 1996; pp. 1259–1263.