Atmospheric rivers have a huge impact on the West Coast’s climate, so scientists are collaborating to understand their patterns.
March 10, 2020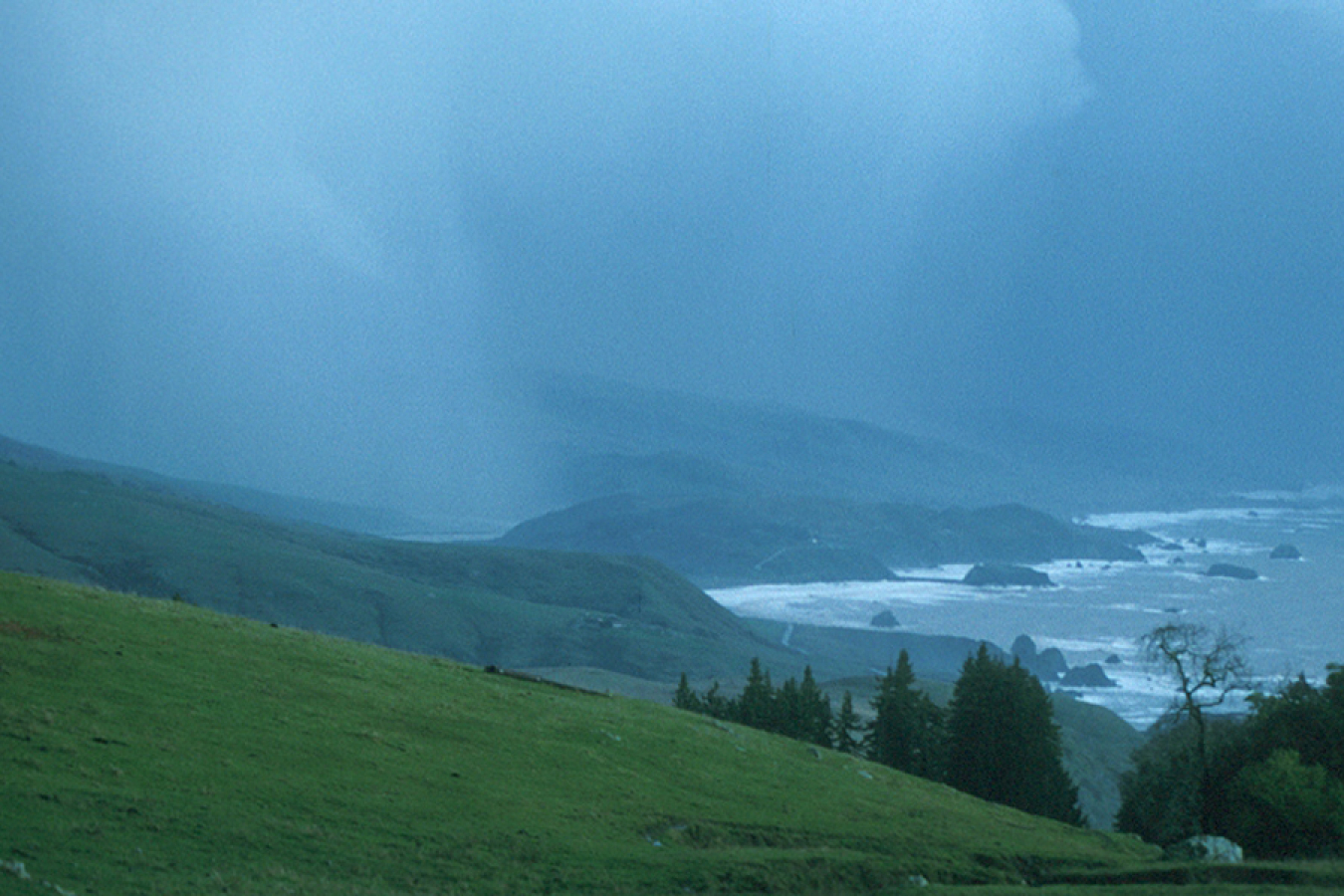
Ice crashed down on the windshield of the small plane. Rain pounded the wings. Water sloshed inside important instruments.
For most pilots, this would be a nightmare.
But still, the plane climbed up through the clouds. It ascended more than 5,000 feet above the ocean.
For this team, these conditions were ideal. In fact, they had been waiting for this moment.
This aircraft, along with three other planes and a research boat, were collecting data on atmospheric rivers—large, narrow sections of the atmosphere that run from the tropics to higher latitudes. Each carries about the same amount of water vapor as liquid water flowing through the mouth of the Amazon River. Upon reaching land—especially coastal areas next to mountains—they jettison much of this moisture. That causes massive amounts of rain and snow to fall.
The vehicles and equipment inside of them were operated by U.S. government agencies to better understand how atmospheric rivers act. The small plane spiraling up through the clouds was run by the Atmospheric Radiation Measurement (ARM) facility, a user facility managed by the Department of Energy’s (DOE) Office of Science. The other aircraft were supported by the U.S. National Oceanic and Atmospheric Administration (NOAA) and the U.S. National Aeronautics and Space Administration (NASA). There was also a research vessel from NOAA and instruments from the National Science Foundation (NSF) and DOE.
Probing observations, satellite data, and climate models, scientists supported by the DOE’s Office of Science are exploring atmospheric rivers’ role in the water and climate cycles. But navigating through the data proved to be trickier than the scientists expected.
Massive Rivers in the Sky
Rivers with liquid water carve a path through the landscape. Similarly, atmospheric rivers have big impacts on their surroundings. Starting over oceans, they’re steered by the westerly winds in the subtropics and extratropics. When they reach land, they smash into the west coasts of continents. The West Coast of the United States, Western Europe, and Western South America all feel their impacts: good and bad.
Upon landfall, atmospheric rivers often bring extreme winter storms. Every time the Russian River in California flooded from 2004 to 2014, an atmospheric river was to blame. These storms can also bring strong winds and follow-on effects like landslides.
But they can also be a savior. Atmospheric rivers’ precipitation provides about 50 percent of the U.S. West Coast’s water supply. Sometimes called “drought-busters,” they ended up to three-quarters of the droughts in the Pacific Northwest from 1950 to 2010.
“Atmospheric rivers are important not only for the short-term, high-impact weather events that are often garnering media attention,” said Jonathan Rutz, an atmospheric scientist at NOAA’s National Weather Service who partners with scientists supported by DOE. “They’re also critically important in the longer term because they provide a lot of the snowpack that turns into the summertime water resources that we need to get us through the year in the West.”
Predicting if an atmospheric river will bring help or disaster—or both—is a major focus of scientists’ efforts. Knowing where and when an atmospheric river will lead to precipitation—as well as how much it will produce—can help water managers and weather forecasters better predict the future.
Pinning down these rivers’ behavior is also essential to improving computer programs that model the climate. At the poles, they’re a major influence on whether huge amounts of snow accumulate or melt.
“Atmospheric rivers are responsible for 90 percent of the polarward moisture transport around the globe,” said Yang Zhou, a postdoctoral researcher at DOE’s Lawrence Berkeley National Laboratory. “Which gives them a very important role in the global hydrological cycle.”
Atmospheric rivers vary a lot from year to year and season to season. As a result, they’re hard to model. Climate change may make them even more unpredictable.
“We may not be able to depend on [the rivers] as much as we have in the past,” said Ashley Payne, an assistant professor of climate and space sciences and engineering at the University of Michigan. Referring to the future, she said, “In addition to changes in atmospheric river intensity, we need to understand how their variability will change.”
A Storm of Data Analysis
There were only a handful of papers in the years after researchers first described atmospheric rivers in the 1990s. But the field exploded in the last decade. In 2015 alone, there were more than 200 scientific papers that mentioned them.
“There were a few studies in the mid-2000s that really caught a lot of attention,” said Ruby Leung, a Battelle fellow at DOE’s Pacific Northwest National Laboratory and the chief scientist on the Energy Exascale Earth System Model (E3SM) project. Those studies underlined both atmospheric rivers’ international nature and big impacts. “It caught the attention of water managers. A much broader community became interested in this.”
Much of the research explores existing satellite data. A recent study by Zhou and her colleague looked at how atmospheric rivers’ initial location over the Pacific Ocean influences their characteristics. The team tracked the life cycle, intensity, and path of every atmospheric river from 1979 to 2019. They found 24 atmospheric rivers hit the West Coast on average each winter. Tracking the rivers’ patterns can help water managers predict where they will appear before they even form.
Other research examines how best to represent and predict atmospheric rivers in computer models. A 2015 study by Leung’s team showed that as scientists increased the spatial detail in the most current Earth system model at the time, the model predicted fewer atmospheric rivers. But that’s not what was happening in real life. The model was overly sensitive because it was having trouble describing where moisture and subtropical winds were moving. This analysis helped scientists improve the model’s accuracy.
Researchers also use models to explore the relationships between atmospheric rivers and their effects. “We’re interested in what kind of large-scale atmospheric conditions are present when atmospheric rivers occur because it’s easier to look at how those conditions change in simulations,” said Naomi Goldenson, a researcher at the University of California, Los Angeles (UCLA). She used climate models to determine the relationship between atmospheric rivers and mountain snowpack in the Northwest U.S. A large number of winter-season atmospheric rivers resulted in more snowpack in the high and cold Sierra Nevada and Rocky Mountains. In contrast, those large numbers of rivers resulted in less snowpack in the wetter, lower Cascade Mountains.
Field observation campaigns allow scientists to discover new insights that aren’t available from existing data. The CalWater 2015 field campaign that ARM participated in was particularly thorough. It provided scientists with a rather unusual view of atmospheric rivers.
“It was quite fun. I am mostly a modeler who usually works in front of a computer,” said Leung, who rode in one of the flights. “That was an experience for me trying to see what I’m actually trying to model with computer code.”
A Flood of Definitions
Even in research, there can be too much of a good thing.
As more researchers dove into studying atmospheric rivers, they discovered there was no quantitative definition. So researchers developed their own. But there are a lot of variables you can choose from. Even the main variable—how much water vapor an atmospheric river is moving per meter per second—can be defined in many ways. The measurement depends on which area within the long, narrow band of moisture one even calls an atmospheric river.
The community ended up with a jumble of definitions. Those resulted in conflicting results. Three separate studies said that atmospheric rivers caused between 15 to 35 percent of California’s precipitation—a huge range. Navigating through the data became challenging.
“I would be talking about an orange and I’d be talking to someone who was talking about an apple, but we were talking about atmospheric rivers,” said Payne. “We had no baseline to compare our results.”
But the differences offered an opportunity to work together.
“It combines the weather and climate communities,” said Goldenson. “We don’t always speak the exact same language or the same priorities or the way we approach the problem. That’s both a challenge and a benefit.”
Chasing Down a Set of Solutions
Tackling the problem, the community launched the Atmospheric River Tracking Method Intercomparison Project (ARTMIP). The project is creating a framework that will help scientists compare methods of identifying and tracking atmospheric rivers. That framework will enable scientists and stakeholders to choose the right method for their application. Scientists have embraced the collaboration.
“It’s a really friendly environment and everyone’s willing to discuss,” said Zhou. “The ARTMIP project is not a competition for the best method.”
So far, the project has tested 20 different approaches on a baseline data set. For each three-hour time period in the data, the project pinpoints if a method says whether or not there is an atmospheric river present along the California coast. Which measurements the techniques used led to big differences in the number of atmospheric rivers they identified.
“As far as I’m aware, there’s nothing else like this that’s ever been done before,” said Rutz.
But they also found that the methods weren’t as different as they first seemed. Most of them measured the same features. Instead, their dissimilarities came from how strict or broad their definition was. Strict definitions mainly identified strong atmospheric rivers. Broad definitions counted weaker ones as well. Each type of model had its own advantages and disadvantages, depending on the situation.
Researchers are already putting these conclusions into practice. A study by Leung and her team examined atmospheric rivers’ characteristics that cause extreme precipitation on the U.S. West Coast. Comparing six different methods identified by ARTMIP, they saw three different types of atmospheric rivers: short-duration and low-intensity ones, short-duration and high-intensity ones, and long-duration ones. The more restrictive methods only identified the high-intensity rivers. As a result, they were better at predicting extreme precipitation. Based on the analysis, the scientists found a way to identify seven to 10 days in advance which atmospheric rivers could cause extreme precipitation on the West Coast.
Now, the ARTMIP group is developing a scale from one to five that categorizes atmospheric rivers. It’s based on the amount of water vapor the rivers transport and their life cycle.
While the new definitions will be helpful, it’s the researchers’ collaboration—whether in the sky or conference rooms—that will allow them to effectively make their way through these rivers.
“It’s bringing together people who are approaching this problem from different angles,” said Goldenson. “I find that to be the most valuable in terms of potential for advancing our understanding even more than just comparing the raw numbers.”
The Office of Science is the single largest supporter of basic research in the physical sciences in the United States and is working to address some of the most pressing challenges of our time. For more information, please visit https://energy.gov/science.
Shannon Brescher Shea
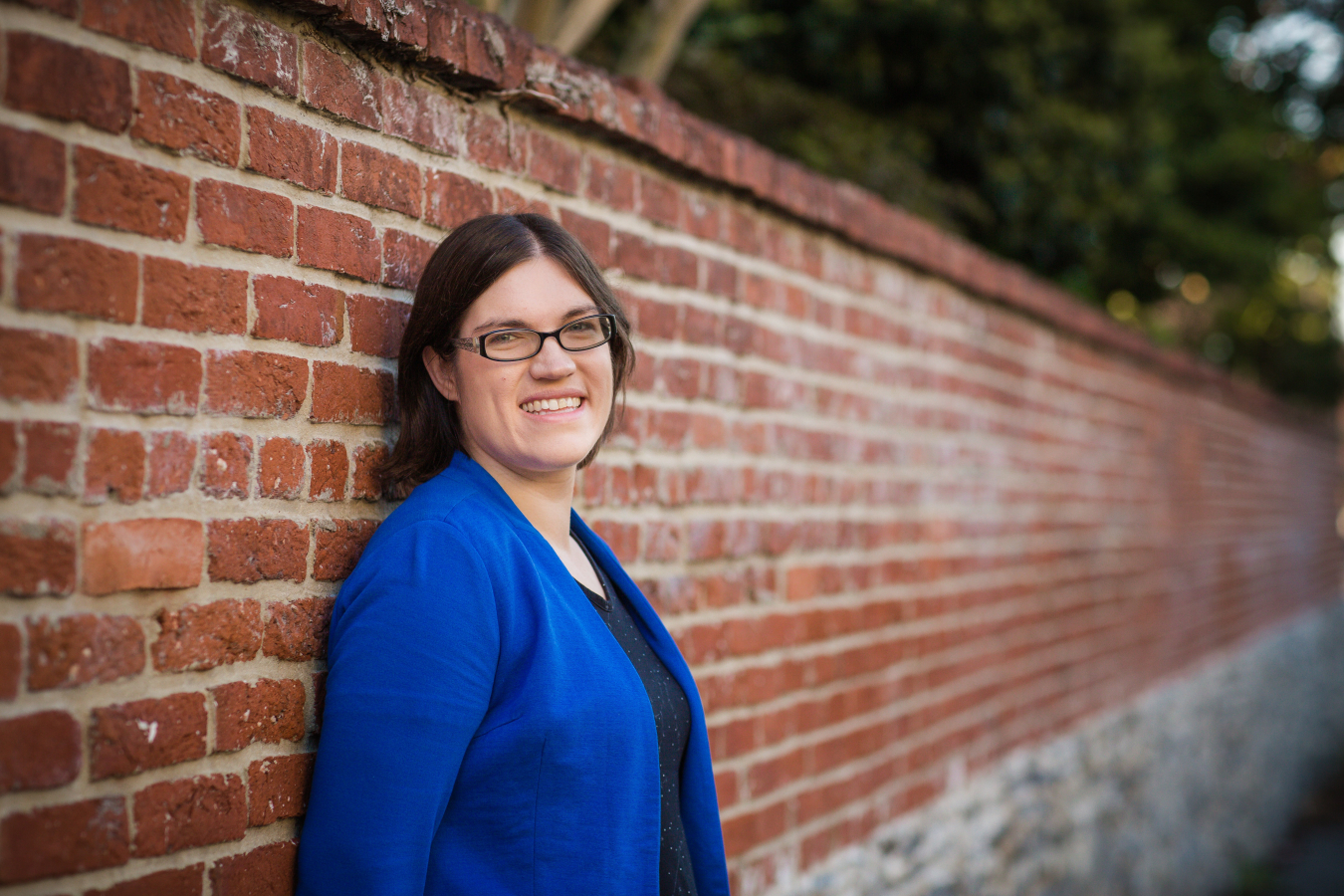
Shannon Brescher Shea (shannon.shea@science.doe.gov) is the social media manager and senior writer/editor in the Office of Science’s Office of Communications and Public Affairs. She writes and curates content for the Office of Science’s Twitter and LinkedIn accounts as well as contributes to the Department of Energy’s overall social media accounts. In addition, she writes and edits feature stories covering the Office of Science’s discovery research and manages the Science Public Outreach Community (SPOC). Previously, she was a communications specialist in the Vehicle Technologies Office in the Office of Energy Efficiency and Renewable Energy. She began at the Energy Department in 2008 as a Presidential Management Fellow. In her free time, she enjoys bicycling, gardening, writing, volunteering, and parenting two awesome kids.