First-ever simulation could lead to vital understanding of wind power plant performance.
Wind Energy Technologies Office
October 13, 2020First-ever simulation could lead to vital understanding of wind power plant performance
Wind power plant performance depends on local environmental conditions—and understanding those conditions is crucial in determining wind power plant performance. Factors such as sloping terrain, surface type and cover, atmospheric heating and cooling, and weather events all play a role in the wind resource and conversion to energy in wind power plants. However, most models used for wind power plant design and operation do not sufficiently account for environmental effects and their impacts on energy production potential. This limits our understanding of wind power plant dynamics and, thus, our ability to design, site, and operate wind power plants for maximum production and reliability.
Scale separation—differences in spatial and temporal scales between diverse atmospheric physical processes—is a key challenge to simulating wind power plants in their natural environments. For example, weather features, such as fronts or pressure systems, cover many kilometers (mesoscale) over minutes to hours. Turbulent flow phenomena that impact wind turbines are much smaller events (microscale) and occur in less than seconds. Capturing these differences in time and space scales over a broad range accurately, and in the same model, is extremely difficult.
“The ability to seamlessly simulate wind plant interactions with the surrounding environment could lead to major advances in wind energy science and operation,” said Lawrence Livermore National Laboratory (LLNL) researcher Robert Arthur. “Predicting the response of wind farms to changing flow conditions would allow us to design and operate them more efficiently.”
To meet this challenge, Arthur and other LLNL researchers partnered with scientists at the National Center for Atmospheric Research; the University of California, Berkeley; the University of Colorado Boulder; and Texas Tech University to develop and demonstrate a novel, mesoscale-to-microscale wind power plant modeling framework. Built within the Weather Research and Forecasting (WRF) model, the framework combines the capability of a weather model to capture realistic meteorological and environmental conditions with the capability of a wind turbine model to capture flow interactions and wakes.
WRF was selected for its open-source code, worldwide user base, and ability to perform multiscale simulations. Additionally, the new framework leverages several WRF model improvements developed and implemented as part of DOE’s Atmosphere to Electrons initiative. These include methods for improved modeling of turbulence across scales as well as an embedded wind turbine model that calculates the lift and drag forces on the turbine blade and the momentum exchanged between the turbine and the passing wind.
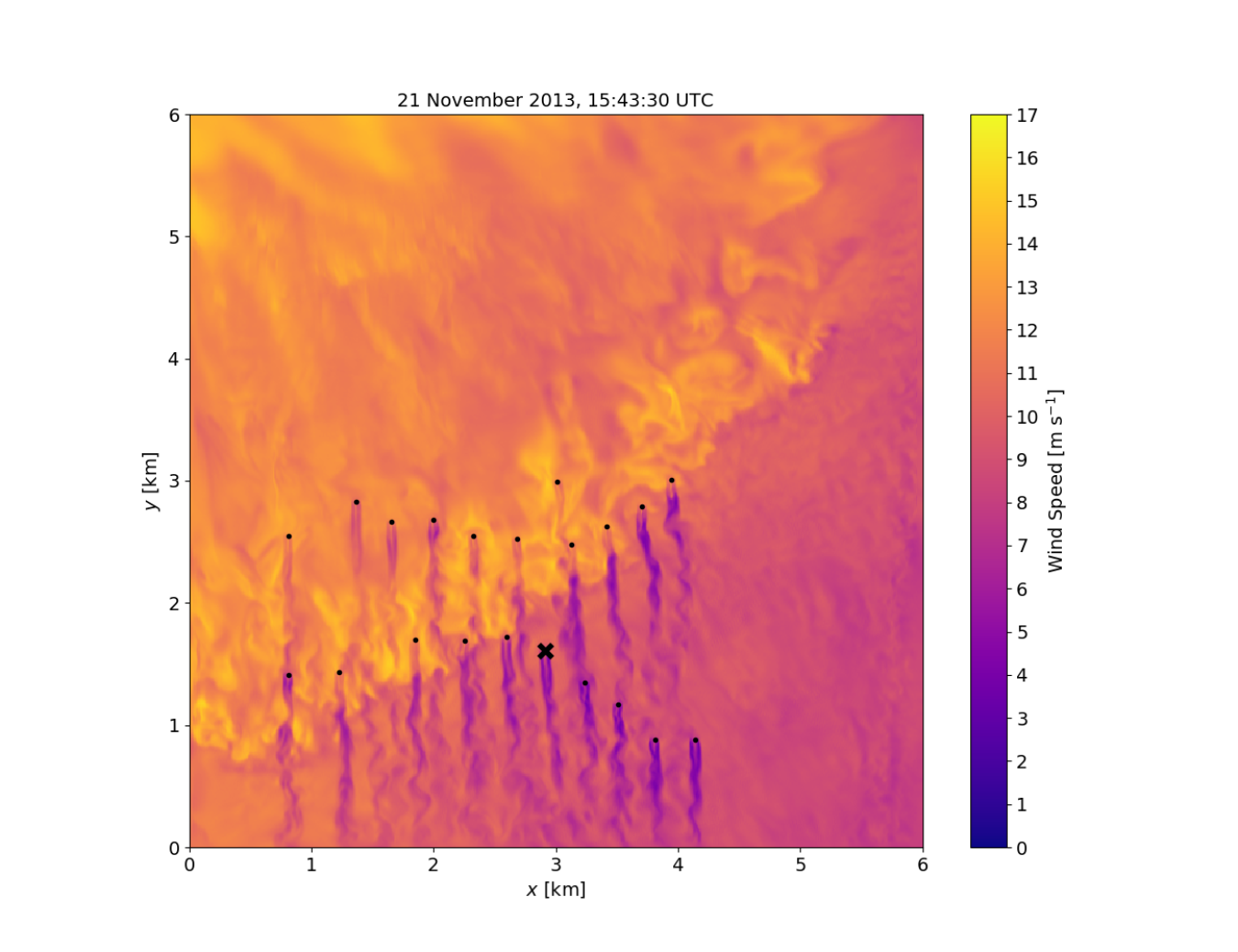
Figure 1. A new wind power plant modeling framework developed by LLNL and partners allows large-scale weather features to interact directly with wind turbines for an improved representation of wakes and turbulence. Image courtesy of Robert Arthur, LLNL
In an article published in the journal Atmosphere, the LLNL-led research team describes applying the new framework to examine a cold front passing through a utility-scale wind power plant in Oklahoma. The study, funded in part by the U.S. Department of Energy’s Wind Energy Technologies Office (WETO), demonstrated for the first time a seamless multiscale simulation of wind power plant performance under complex atmospheric conditions.
The study compared model output to radar measurements of the full, three-dimensional wind field within the plant as the cold front passes through. The model captures the dynamics of the frontal passage, including an abrupt increase in wind speed, change in wind direction, and increase in turbulence intensity. Additionally, the modeled turbines respond to the passing front by yawing (rotating) into the predominant wind direction, leading to an accurate representation of the wake structure (Figure 1 and associated animation). By capturing both the atmospheric dynamics and turbine behavior, power output is also well-predicted by the model (Figure 2).
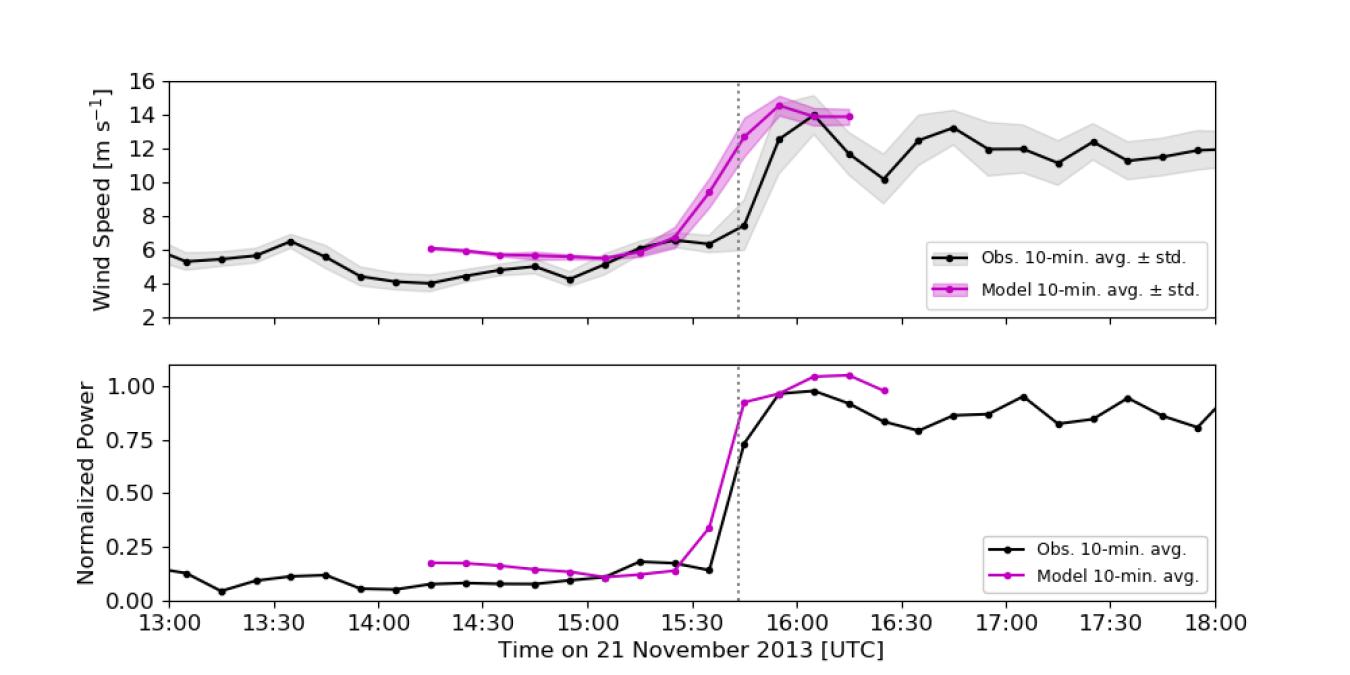
Figure 2. The new framework enables accurate calculations of wind plant power output in response to complex environmental conditions. Figure courtesy of Robert Arthur, LLNL
“The rapidly changing nature of this event would make it quite difficult to capture in a traditional wind turbine modeling framework,” Arthur said. “But our new model captured both the dynamics of the passing weather front and the turbines’ response.”
Based on the promising results of this study, the new modeling framework is slated for further development and use in several upcoming DOE wind projects. These include the Wind Forecast Improvement Project 3 and American Wake Experiment, both of which involve extensive observational campaigns that will provide valuable data for model validation.
Explore previous editions of the Wind R&D Newsletter or browse articles by topic: