Building on its role as a world-class research facility, DOE's National Wind Technology Center at NREL—along with CREE, DNV KEMA, GE Wind, Romax Technology, Vattenfall Windpower, and DOE's Oak Ridge National Laboratory—developed an innovati...
Wind Energy Technologies Office
October 1, 2013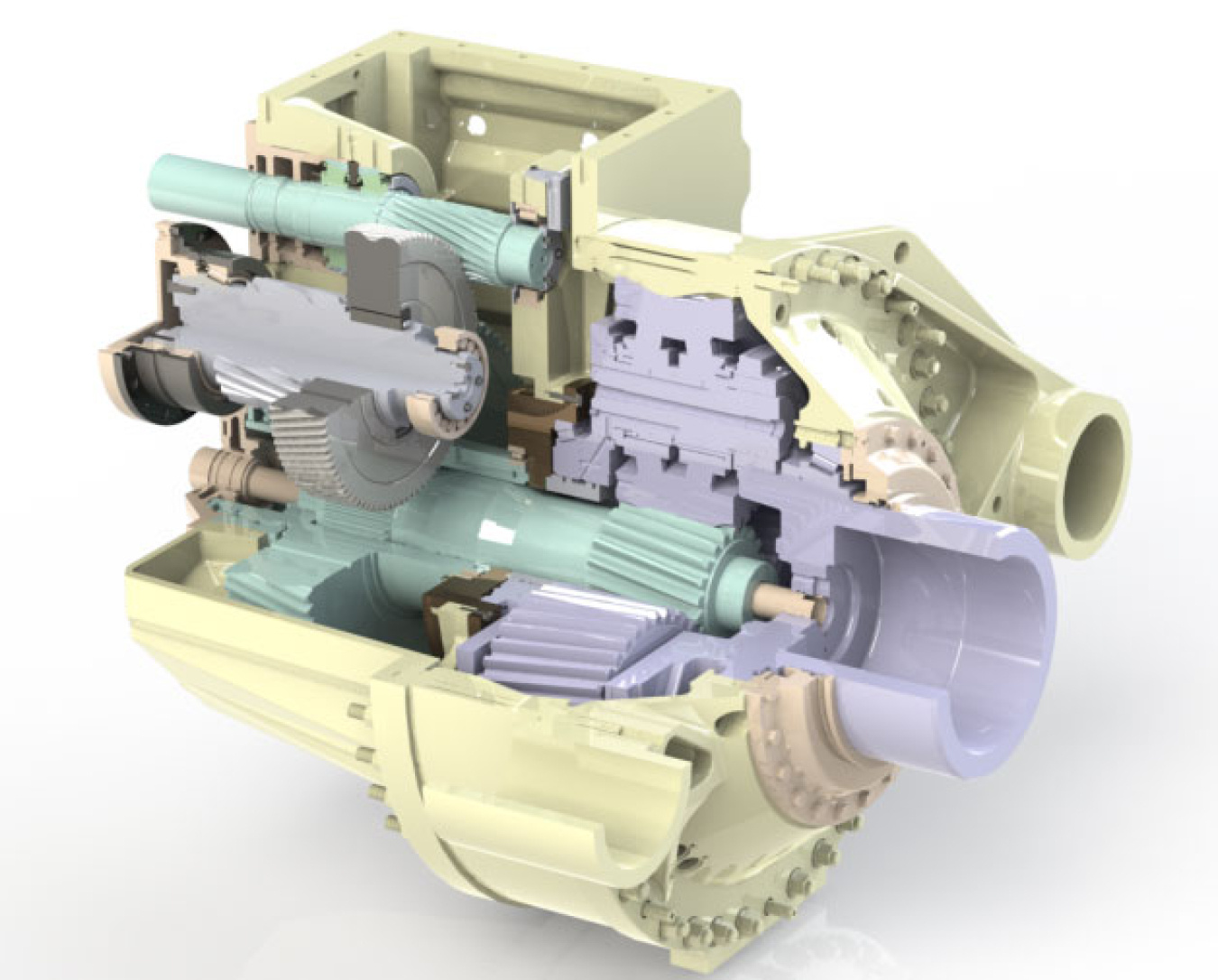
The innovative drivetrain designed by NREL and its partners applies a system approach to improve all three major components: a single-stage gearbox, a medium-speed permanent-magnet generator, and a high-efficiency power converter.
Illustration by Romax Technology
Building on its role as a world-class research facility, the U.S. Department of Energy's (DOE's) National Wind Technology Center (NWTC) at the National Renewable Energy Laboratory (NREL)—along with CREE, DNV KEMA, GE Wind, Romax Technology, Vattenfall Windpower, and DOE's Oak Ridge National Laboratory—developed an innovative, medium-speed, medium-voltage wind turbine drivetrain design. Tapping into unparalleled expertise, the team created a drivetrain that can increase reliability, decrease mass, improve efficiency, and reduce the cost of wind energy. In addition, the drivetrain design can scale up to ratings as high as 10 megawatts (MW) while maintaining the lowest possible costs.
In 2011, six teams were awarded by DOE to conduct a phase one study of advanced drivetrain technologies that could be scaled to larger turbines and significantly reduce the cost of wind energy. From these studies, the NWTC's team and Advanced Magnet Lab were selected for phase two awards, which were intended to demonstrate the technologies' commercialization potential.
The NWTC team's design concept applies a system approach to improve the conventional wind turbine drivetrain, focusing on all three of its major components: a single-stage gearbox, a medium-speed permanent-magnet generator, and a high-efficiency power converter. Traditional three-stage high-speed gearbox designs have been plagued with reliability issues caused by the large and unpredictable loads imparted on the gears and bearings by the wind acting on the rotor and by utility faults acting through the generator. Therefore, the team's gearbox design consists of a single planetary stage that uses compliant flex-pins and journal bearings to support the planets, eliminating the lower-reliability, higher-speed stages. This new configuration improves the load distribution and increases the drivetrain's overall reliability. In addition, the single-stage gearbox connects to a medium-speed generator that uses a fraction of the rare-earth magnets typically used in direct-drive permanent-magnet generators of similar power.
Historically, wind plants have been subjected to less stringent utility interconnection requirements than fossil-fuel plants. However, because of the success and substantial growth of wind energy in recent years, wind plant interconnection requirements are now the same as fossil-fuel plants. As a result, these requirements drive the design of new power converters.
The team's power converter design incorporates innovative software algorithms that are grid-friendly, compliant with emerging requirements, and support the continued growth of wind power as a large contributor to power generation. The NWTC team will also be exploring medium-voltage, wide-band gap, silicon-carbide power modules. These state-of-the-art power modules are expected to significantly reduce the losses within the power converter, leading to increased efficiency, energy capture, and revenue.
In phase two, the team will design, fabricate, and test a drivetrain that incorporates these advancements. The drivetrain elements will be tested in the 2.5-MW dynamometer and newly commissioned controllable grid interface at the NWTC and on a specialized, medium-voltage power electronic test rig. Upon the successful completion of testing, technology readiness levels will be advanced and combined with a commercialization plan that will lead to global deployments of the drivetrain technologies. The team's designs will further reduce the cost of wind energy and ensure that U.S. companies are at the forefront of technical innovation within the global wind energy industry.